تاريخ الفيزياء
علماء الفيزياء
الفيزياء الكلاسيكية
الميكانيك
الديناميكا الحرارية
الكهربائية والمغناطيسية
الكهربائية
المغناطيسية
الكهرومغناطيسية
علم البصريات
تاريخ علم البصريات
الضوء
مواضيع عامة في علم البصريات
الصوت
الفيزياء الحديثة
النظرية النسبية
النظرية النسبية الخاصة
النظرية النسبية العامة
مواضيع عامة في النظرية النسبية
ميكانيكا الكم
الفيزياء الذرية
الفيزياء الجزيئية
الفيزياء النووية
مواضيع عامة في الفيزياء النووية
النشاط الاشعاعي
فيزياء الحالة الصلبة
الموصلات
أشباه الموصلات
العوازل
مواضيع عامة في الفيزياء الصلبة
فيزياء الجوامد
الليزر
أنواع الليزر
بعض تطبيقات الليزر
مواضيع عامة في الليزر
علم الفلك
تاريخ وعلماء علم الفلك
الثقوب السوداء
المجموعة الشمسية
الشمس
كوكب عطارد
كوكب الزهرة
كوكب الأرض
كوكب المريخ
كوكب المشتري
كوكب زحل
كوكب أورانوس
كوكب نبتون
كوكب بلوتو
القمر
كواكب ومواضيع اخرى
مواضيع عامة في علم الفلك
النجوم
البلازما
الألكترونيات
خواص المادة
الطاقة البديلة
الطاقة الشمسية
مواضيع عامة في الطاقة البديلة
المد والجزر
فيزياء الجسيمات
الفيزياء والعلوم الأخرى
الفيزياء الكيميائية
الفيزياء الرياضية
الفيزياء الحيوية
الفيزياء العامة
مواضيع عامة في الفيزياء
تجارب فيزيائية
مصطلحات وتعاريف فيزيائية
وحدات القياس الفيزيائية
طرائف الفيزياء
مواضيع اخرى
NEUTRINOS
المؤلف:
E. R. Huggins
المصدر:
Physics 2000
الجزء والصفحة:
330
26-11-2020
2356
NEUTRINOS
The discovery of the neutrino, or at least the prediction of its existence, is another important event in the history of physics that is related to the conservation of energy and linear momentum. After Rutherford’s discovery of the nucleus, it became clear that the high energy radioactive emissions, α, β, and γ rays, must be coming from the nucleus of the atom. Thus a study of these rays should give valuable information about the nature of the nucleus itself.
After a number of years of experimentation it was determined that whenever an α particle or γ ray was emitted, the energy carried out by the α particle or γ ray was precisely equal to the energy lost by the nucleus.
But decays involving β particles were different. In studying β decays, one always got a spread of energies of the β particle. Sometimes the β particle carried out almost all the energy lost by the nucleus, and sometimes only the relatively small rest energy of the electron. By the late 1920s it was clear that energy was apparently not conserved in β decay reactions. Neils Bohr proposed that the law of conservation of energy had to be modified for nuclear reactions involving β decays. The new rule was that the final energy was always less than or equal to the initial energy. In 1930 Wolfgang Pauli, one of the founders of quantum theory, objected to the idea that energy was not conserved in β decay events. Pauli noted that the conservation of energy, linear momentum, and angular momentum are all apparently violated at the same time in β decay. Either the entire structure of physical law was being violated, or there was another explanation. Pauli’s other explanation was that the energy, the linear momentum, and the angular momentum were all being carried out at the same time by an unseen particle.
If Pauli’s particle existed, it would need the following properties: (1) it had to be electrically neutral because no electric charge was lost in β decays; (2) it would have to have a very small rest mass because the electron or β particle sometimes carried out almost all the available energy, leaving none for creating the new particle’s rest mass; (3) the new particle had to have almost no interaction with matter, otherwise someone would have seen it. Initially there was not much enthusiasm for Pauli’s idea of an undetectable particle. At that point no one had seen an electrically neutral particle, and the fact that it did not interact with matter made it seem too speculative.
In 1932 the neutron was discovered which demonstrated that neutral particles did exist. Shortly after that Enrico Fermi developed a detailed theory of the weak interaction in which neutrinos played a significant role. (Fermi called Paul’s particle the neutrino, or “little neutral one” to distinguish it from the more massive neutron.) Detailed verification of Fermi’s weak interaction theory convinced the physics community that neutrinos should exist.
The neutrino, actually detected in 1956 and now commonly seen in numerous experiments, is a remarkable particle in that it is subject to only the weak and gravitational interactions. All other known particles are subject to the electric and nuclear forces. For example π mesons are subject to the nuclear force, and can travel only a short distance through matter before colliding with a nucleus and being stopped.
The muon discussed in the relativity chapter, does not feel the strong nuclear force and therefore can travel much farther through matter (hundreds of meters) before being stopped. Muons are electrically charged and therefore are stopped by the weaker electric interaction. Photons also interact through the electric interaction, and therefore have a limited range traveling through matter. (An X-ray is an example of a photon passing through matter.)
The weak interaction is so weak compared to the nuclear or electric force, that a particle like the neutrino which feels only the weak interaction force, can travel incredible distances through matter before being stopped. To have a good chance of stopping a single neutrino with a stack of lead, one would need a pile of lead, light years thick.
This does not mean that neutrinos are impossible to detect. Instead of using a detector light years across to detect one neutrino, one can use a source that produces an incredible number of neutrinos and use a reasonable sized detector so that one has some chance of stopping a few neutrinos. In 1956 Cowan and Rines placed a tank car full of carbon tetrachloride cleaning fluid in front of a nuclear reactor that was estimated to emit about 1015 neutrinos per square centimeter per second. They observed that about two chlorine atoms per month in the tank car of carbon tetrachloride were converted by neutrino interactions into argon atoms which were counted individually.
In modern experiments carried out using high energy particle accelerators, neutrino reactions are routinely seen. The reason that more neutrinos are detected in these experiments is that the weak interaction becomes less weak as the energy of the particles involved increases. The high energy accelerators produce neutrinos with great enough energy that they are not too difficult to detect. As a result, the neutrino interactions has become an important research tool in the study of the basic interactions of matter. Neutrinos make a particularly clean tool for these studies because they have no nuclear or electric interactions. Neutrino experiments are not contaminated by effects of the nuclear and electric forces.
الاكثر قراءة في مواضيع عامة في الفيزياء النووية
اخر الاخبار
اخبار العتبة العباسية المقدسة
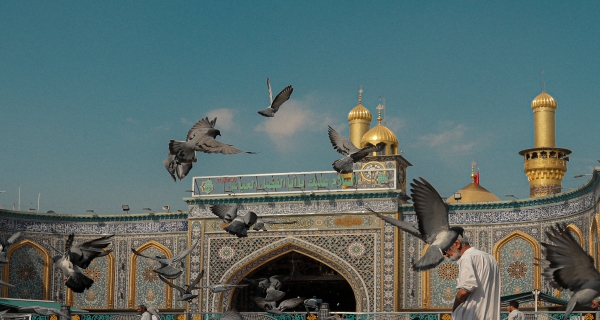
الآخبار الصحية
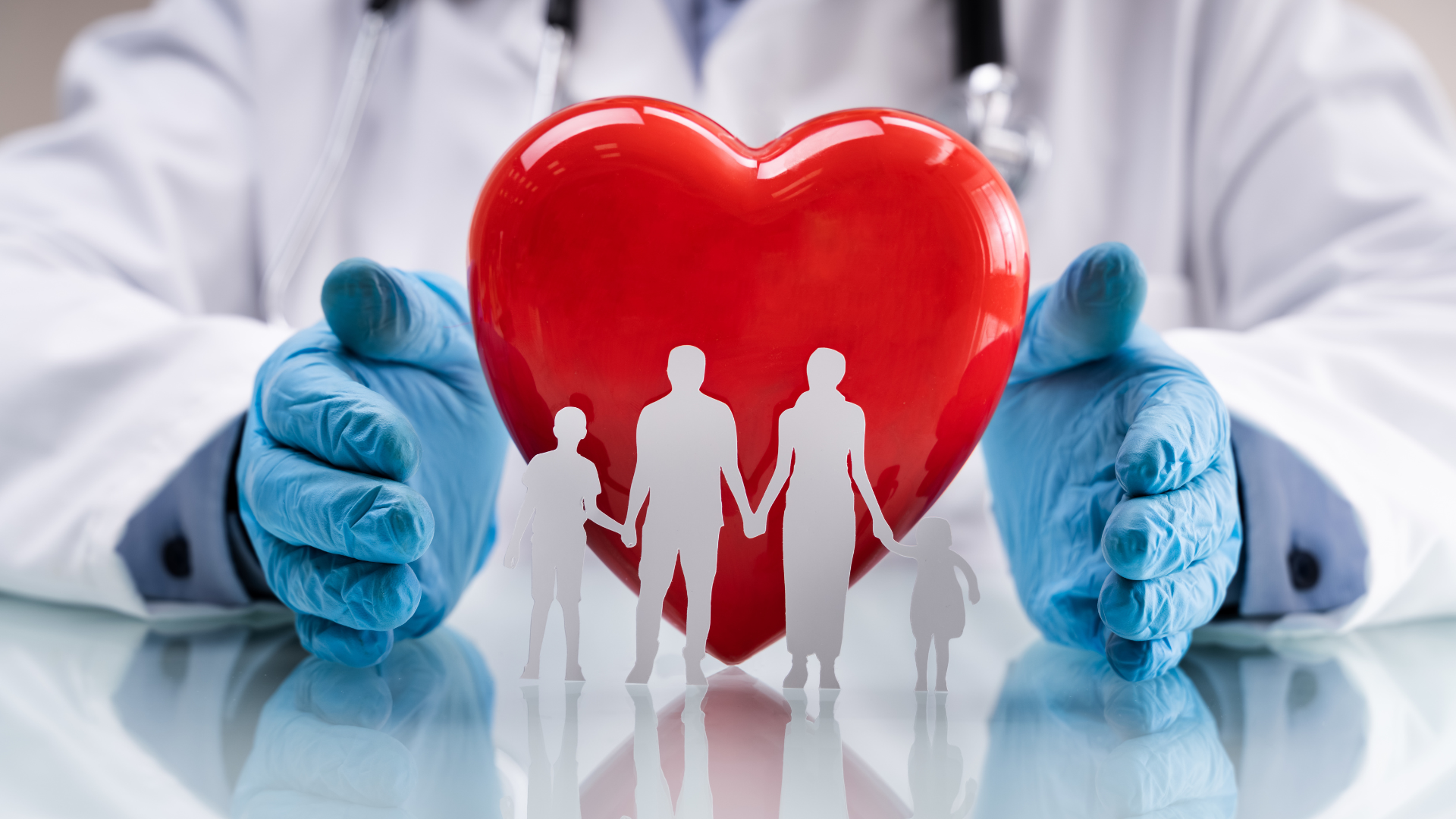