النبات
مواضيع عامة في علم النبات
الجذور - السيقان - الأوراق
النباتات الوعائية واللاوعائية
البذور (مغطاة البذور - عاريات البذور)
الطحالب
النباتات الطبية
الحيوان
مواضيع عامة في علم الحيوان
علم التشريح
التنوع الإحيائي
البايلوجيا الخلوية
الأحياء المجهرية
البكتيريا
الفطريات
الطفيليات
الفايروسات
علم الأمراض
الاورام
الامراض الوراثية
الامراض المناعية
الامراض المدارية
اضطرابات الدورة الدموية
مواضيع عامة في علم الامراض
الحشرات
التقانة الإحيائية
مواضيع عامة في التقانة الإحيائية
التقنية الحيوية المكروبية
التقنية الحيوية والميكروبات
الفعاليات الحيوية
وراثة الاحياء المجهرية
تصنيف الاحياء المجهرية
الاحياء المجهرية في الطبيعة
أيض الاجهاد
التقنية الحيوية والبيئة
التقنية الحيوية والطب
التقنية الحيوية والزراعة
التقنية الحيوية والصناعة
التقنية الحيوية والطاقة
البحار والطحالب الصغيرة
عزل البروتين
هندسة الجينات
التقنية الحياتية النانوية
مفاهيم التقنية الحيوية النانوية
التراكيب النانوية والمجاهر المستخدمة في رؤيتها
تصنيع وتخليق المواد النانوية
تطبيقات التقنية النانوية والحيوية النانوية
الرقائق والمتحسسات الحيوية
المصفوفات المجهرية وحاسوب الدنا
اللقاحات
البيئة والتلوث
علم الأجنة
اعضاء التكاثر وتشكل الاعراس
الاخصاب
التشطر
العصيبة وتشكل الجسيدات
تشكل اللواحق الجنينية
تكون المعيدة وظهور الطبقات الجنينية
مقدمة لعلم الاجنة
الأحياء الجزيئي
مواضيع عامة في الاحياء الجزيئي
علم وظائف الأعضاء
الغدد
مواضيع عامة في الغدد
الغدد الصم و هرموناتها
الجسم تحت السريري
الغدة النخامية
الغدة الكظرية
الغدة التناسلية
الغدة الدرقية والجار الدرقية
الغدة البنكرياسية
الغدة الصنوبرية
مواضيع عامة في علم وظائف الاعضاء
الخلية الحيوانية
الجهاز العصبي
أعضاء الحس
الجهاز العضلي
السوائل الجسمية
الجهاز الدوري والليمف
الجهاز التنفسي
الجهاز الهضمي
الجهاز البولي
المضادات الحيوية
مواضيع عامة في المضادات الحيوية
مضادات البكتيريا
مضادات الفطريات
مضادات الطفيليات
مضادات الفايروسات
علم الخلية
الوراثة
الأحياء العامة
المناعة
التحليلات المرضية
الكيمياء الحيوية
مواضيع متنوعة أخرى
الانزيمات
Ferredoxins
المؤلف:
D. B. Knaff
المصدر:
In Oxygenic Photosynthesis: The Light Reactions
الجزء والصفحة:
9-5-2016
2524
Ferredoxins
Ferredoxins (Fds) are simple iron–sulfur proteins; that is, they do not contain other prosthetic groups beside one or two iron-sulfur (FeS) clusters. The first Fd to be discovered was that from Clostridium pasteurianum in 1962, immediately followed by the one from plant chloroplasts (1). Fds are ubiquitous, small, generally very acidic proteins, diverse in structure and function, that are involved mainly as electron carriers of low oxidation/reduction potential in fundamental metabolic processes like photosynthesis, nitrogen fixation, and assimilation of hydrogen, nitrogen, and sulfur. Recently, functions other than electron transfer have been discovered for some members of the very large class of FeS proteins. Indeed, it has become clear that most bacteria and plants contain not just one Fd, but a variety, distinguished by amino acid sequence, FeS cluster type, redox potential, and function. The Archaebacteria are particularly rich in Fds: Methanococcus jannaschii has 8 Fds and 6 polyFds, and Archeoglobus fulgidus has 8 Fds; as are the diazotroph bacteria (there are 8 to 9 Fds in Azotobacter vinelandii), followed by higher plants, from which at least six soluble Fds have been isolated. In bacteria, the number of Fds varies widely: just one Fd in Bacillus subtilis, but four in both Mycobacterium tubercolosis and Escherichia coli. In the first completed eukaryotic genome, that of the yeast Saccharomyces cerevisiae, only one Fd is present, which is similar to Fds found in mammalian mitochondria (adrenodoxin/renodoxin) that function in the hydroxylations catalyzed by cytochrome P450 for formation of steroid hormones, vitamin D metabolites, and bile acids.
Fds contain iron and sulfur atoms, organized in three different types of iron–sulfur clusters that are defined as 2Fe–2 S, 4Fe–4 S, and 3Fe–4 S. These proteins absorb light in the 300- to 500-nm wavelength region: their solutions are brownish in color and become paler upon reduction. Typical electron para-magnetic resonance signals are shown by Fds in either the oxidized or reduced states. These proteins are sensitive to acids, oxidants, mercurials, and alkylating agents, but they show fair stability in the pH range 6 to 9 and to heat denaturation in the absence of oxygen. Fds are best divided into two classes: 2Fe–2 S Fds and 4Fe–4 S Fds, that may include the 3Fe–4 S clusters.
1. 2Fe–2 S Ferredoxins
These Fds are ubiquitous hydrophilic proteins of 11 to 15 kDa containing one 2Fe–2 S cluster that function as one-electron carriers. The iron-binding amino-acid sequence motif is Cys–X4–Cys–X2–Cys, which provides three of the four Cys ligands to the cluster; the fourth Cys ligand is distant in the primary structure. In some bacterial Fds of the hydroxylating dioxygenases (2) and in adrenodoxin, the spacing between the two first Cys residues is X3 and X5, respectively. The reduction potential varies in the range –400 to –100 mV (relative to the hydrogen electrode). The prototype of this class is the plant-type Fd (3), which has the role of distributing electrons received from the photoreduced photosystem I to several ferredoxin-dependent enzymes. The protein structure motif characteristic of these proteins is called “b-grasp” (a five-stranded b-sheet with an a-helix lying on top of the sheet). Adrenodoxin and putidaredoxin have additional small interaction domains.
2. 4Fe–4 S and 3Fe–4 S Ferredoxins
The prototype bacterial ferredoxin (4, 5) contains FeS clusters of the so-called “cubane” type, in which the four iron and four sulfide atoms are placed alternatively on the corners of a somewhat distorted cube. Coordination to the protein typically occurs through four cysteine thiolate groups to the four iron atoms. The most common bacterial ferredoxins contain two such clusters in a polypeptide chain of 50 to 60 residues. These proteins are typically found in anaerobes, where they are involved in one-electron transfer in low-potential systems, collecting reducing equivalents from a wide variety of substrates and metabolic cycles. A structural distinction has been made between the above classes of ferredoxins, which have been divided into “clostridial-type” and “photosynthetic bacterial and nif-related” ferredoxins. The former contain two Cys–X2–Cys–X2–Cys–X3–Cys–Pro motifs, while the latter contain one motif of that type and the more unusual Cys–X2–Cys–X 7–9–Cys–X3–Cys–Pro motif. All these proteins are low-potential electron carriers, with typical reduction potential in the –390 to –450 mV range.
Some of the clostridial-type ferredoxins found in Desulfovibrio spp and in extreme thermophiles such as Pyrococcus furiosus contain a Cys–X2–Asp–X2–Cys binding motif, with a remote Cys residue as the fourth ligand and the Asp available to take the place of the missing central Cys residue of this sequence. These proteins contain a 3Fe–4 S cluster, which can be converted reversibly to a 4Fe–4 S cluster upon addition of iron and which may bind to exogenous ligands, such as thiol groups and cyanide. Cornerless cubanes containing the 3Fe–4 S clusters may be formed upon oxidation (by oxygen or ferricyanide) of regular 4Fe–4 S clusters, in a possibly reversible process.
References
1. D. I. Arnon (1988) Trends Biochem. Sci. 13, 30–33.
2. J. R. Mason and R. Cammack (1992) Annu. Rev. Microbiol . 46, 277–305.
3. D. B. Knaff (1996) In Oxygenic Photosynthesis: The Light Reactions (D. R. Ort and C. F. Yocum, eds.), Kluwer, The Netherlands, pp. 333–361.
4. M. Bruschi and F. Guerlesquin (1988) FEMS Microbiol. Rev. 54, 155–176.
5. J. J. G. Moura, A. L. Macedo, and P. N. Palma (1994) Methods Enzymol. 243, 165–188.
الاكثر قراءة في مواضيع عامة في الاحياء الجزيئي
اخر الاخبار
اخبار العتبة العباسية المقدسة
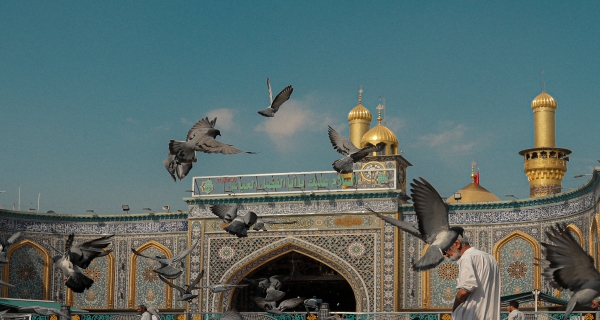
الآخبار الصحية
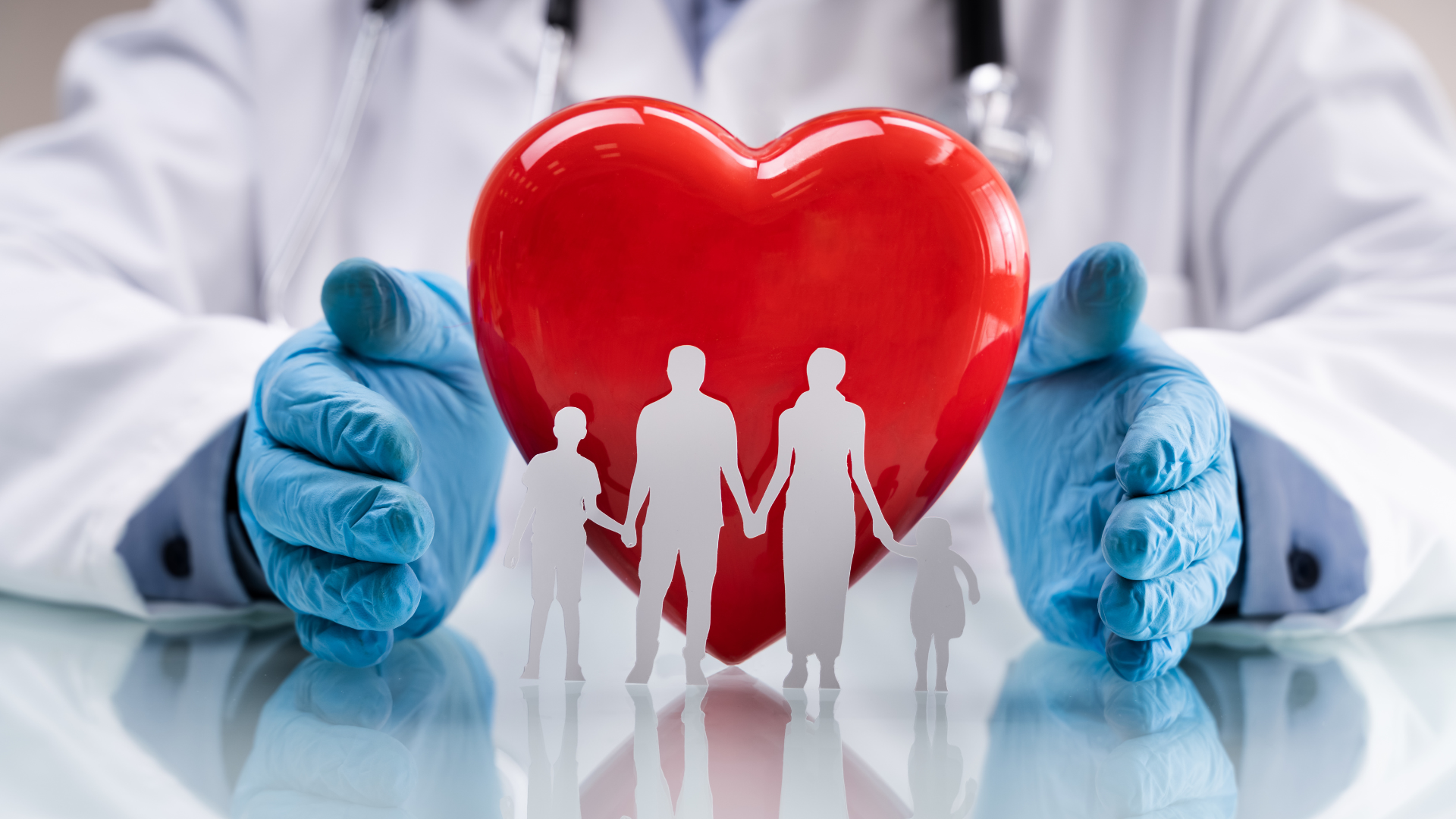