النبات
مواضيع عامة في علم النبات
الجذور - السيقان - الأوراق
النباتات الوعائية واللاوعائية
البذور (مغطاة البذور - عاريات البذور)
الطحالب
النباتات الطبية
الحيوان
مواضيع عامة في علم الحيوان
علم التشريح
التنوع الإحيائي
البايلوجيا الخلوية
الأحياء المجهرية
البكتيريا
الفطريات
الطفيليات
الفايروسات
علم الأمراض
الاورام
الامراض الوراثية
الامراض المناعية
الامراض المدارية
اضطرابات الدورة الدموية
مواضيع عامة في علم الامراض
الحشرات
التقانة الإحيائية
مواضيع عامة في التقانة الإحيائية
التقنية الحيوية المكروبية
التقنية الحيوية والميكروبات
الفعاليات الحيوية
وراثة الاحياء المجهرية
تصنيف الاحياء المجهرية
الاحياء المجهرية في الطبيعة
أيض الاجهاد
التقنية الحيوية والبيئة
التقنية الحيوية والطب
التقنية الحيوية والزراعة
التقنية الحيوية والصناعة
التقنية الحيوية والطاقة
البحار والطحالب الصغيرة
عزل البروتين
هندسة الجينات
التقنية الحياتية النانوية
مفاهيم التقنية الحيوية النانوية
التراكيب النانوية والمجاهر المستخدمة في رؤيتها
تصنيع وتخليق المواد النانوية
تطبيقات التقنية النانوية والحيوية النانوية
الرقائق والمتحسسات الحيوية
المصفوفات المجهرية وحاسوب الدنا
اللقاحات
البيئة والتلوث
علم الأجنة
اعضاء التكاثر وتشكل الاعراس
الاخصاب
التشطر
العصيبة وتشكل الجسيدات
تشكل اللواحق الجنينية
تكون المعيدة وظهور الطبقات الجنينية
مقدمة لعلم الاجنة
الأحياء الجزيئي
مواضيع عامة في الاحياء الجزيئي
علم وظائف الأعضاء
الغدد
مواضيع عامة في الغدد
الغدد الصم و هرموناتها
الجسم تحت السريري
الغدة النخامية
الغدة الكظرية
الغدة التناسلية
الغدة الدرقية والجار الدرقية
الغدة البنكرياسية
الغدة الصنوبرية
مواضيع عامة في علم وظائف الاعضاء
الخلية الحيوانية
الجهاز العصبي
أعضاء الحس
الجهاز العضلي
السوائل الجسمية
الجهاز الدوري والليمف
الجهاز التنفسي
الجهاز الهضمي
الجهاز البولي
المضادات الحيوية
مواضيع عامة في المضادات الحيوية
مضادات البكتيريا
مضادات الفطريات
مضادات الطفيليات
مضادات الفايروسات
علم الخلية
الوراثة
الأحياء العامة
المناعة
التحليلات المرضية
الكيمياء الحيوية
مواضيع متنوعة أخرى
الانزيمات
Epigenetics
المؤلف:
R. J. Richards
المصدر:
The Meaning of Evolution
الجزء والصفحة:
4-5-2016
2465
Epigenetics
Epigenetics is derived from the Greek word epigenesis, which describes the Aristotelian concept that an organism develops progressively through a series of causal interactions of various parts. During the 16th and 17th centuries, the epigenetic mode of development was held in contrast to the preformation theory, which maintained that development occurred by simple enlargement of a preformed homunculus in the germ cell. More modern definitions include the concept that, during development, cells progress through microenvironments that induce a stepwise restriction of their developmental potentialities into specialized ones via changes in gene expression (2). Based on this view of development, Holliday has defined epigenetics as the study of the mechanisms “that impart temporal and spatial control on the activity of all those genes required for the development of a complex organism from the zygote to the fully formed adult” (3). More recent usage, however, has tended to emphasize the “epi” or “beyond” aspect of the term. Strohman points out that “epigenesis is the historical alternative to genetic determinism and that it establishes the basis for a level of organizational control above the genome” (4). Accordingly, molecular geneticists use the term epigenetics for a process by which the state of gene expression is modified at a given time of development and becomes heritable at the cellular or organismal level. Molecular mechanisms underlying epigenetic phenomena are known only in a few cases. The known mechanisms include modifications of DNA and changes in the chromatin structure, which are thought to be propagated through templating (5).
There exist a broad variety of epigenetic phenomena in many organisms. Perhaps one of the best-studied epigenetic phenomena is mating-type silencing in the yeast Saccharomyces cerevisiae (6, 7). Yeast cells possess one of two mating types, a or a, determined by which of the alternate mating-type alleles is present at the mating-type locus (MAT). In addition, cells harbor two other copies of mating-type genes at the loci called HML and HMR. Despite their identical sequences, the mating-type gene located at the MAT locus is active in transcription; those at HML and HMR are silent. The molecular basis of mating-type silencing appears to lie in the specific type of chromatin structure at the HML and HMR loci.
In the fruit fly, Drosophila melanogaster, several phenomena have been described as epigenetic. The first example, known as position effect variegation (PEV), is observed when a chromosomal segment of euchromatin is translocated adjacent to a heterochromatic region (8). Such a translocation results in variegated spreading of repression from the heterochromatic region into the euchromatic segment, causing a metastable state of expression of the translocated genes. Because the variable spreading of gene inactivation is inherited clonally, the PEV phenomenon is considered epigenetic. The second example includes the silencing of homeotic genes during development (9). Homeotic genes are expressed in specific domains of the body and silenced in others. This spatially regulated silencing is established in early embryogenesis and is maintained throughout development by a group of chromatin-associated proteins known as the Polycomb group proteins.
In mammals, genomic imprinting and X-Chromosome Inactivation in females are both examples of epigenetic effects. Genomic imprinting has been observed for some 20 genes whose expression in an early embryo depends on whether the allele is derived maternally or paternally (10). Some genes are inactive when derived from maternal gametes; in other genes those derived paternally are inactive. The molecular basis of imprinting is known to be methylation of the DNA. The state of inactivity is inherited clonally through subsequent cell divisions by templating of the methylated state. Particular CpG sequences in imprinted genes are methylated in gametes of one sex but not the other. The zygote therefore becomes heterozygous at imprinted genes, carrying a methylated allele and a nonmethylated allele. During development, methylated alleles are not expressed, while homologous copies that are nonmethylated are expressed and provide the necessary functions. In primordial germ cells (the precursors of eggs and sperms), imprinting is erased by demethylation of appropriate residues in the imprinted genes. These residues are remethylated de novo in gametes in either the maternal or paternal mode.
In female mammals, one of two X chromosomes is inactivated, appearing cytologically as a Barr body. The X chromosome is inactivated by coating with the so-called Xist RNA (11). The Xist gene on the active chromosome is turned off by methylation of its promoter, while the Xist gene on the chromosome to be inactivated produces Xist RNA that coats its own chromosome. Once an X chromosome is inactivated, its inactive state is clonally inherited in its descendant cells, without the need of Xist RNA. Thus, Xist RNA appears to initiate an epigenetic state that is perpetuated, without playing a further role.
Lesser known, but equally fascinating, epigenetic phenomena are found in fungi and plants. Neurospora crassa and Ascobolus immersus seem to protect their genomes from assaults by transposable elements through mechanisms known as repeat induced-point mutation (RIP) and methylation-induced premeiotically (MIP), respectively (12, 13). Transposable elements, often present in multiple copies, trigger their mutual silencing in a process known as quelling by methylation or C to T transition mutations of repeated sequences. A paramutation, found in higher plants such as maize, tomato, or tobacco, is a mitotically and meiotically heritable change in gene expression that does not involve alterations in DNA sequences (14). At paramutable loci, some alleles, called paramutable alleles, are susceptible to paramutation, others, known as paramutagenic alleles, induce paramutation in the homologous copy of the same gene.
References
1. R. J. Richards (1992) The Meaning of Evolution, The University of Chicago Press, Chicago, pp. 5-16.
2. C. H. Waddington (1956) Principles of Embryology, Allen and Unwin, London, p. 350.
3. R. Holliday (1990) Phil. Trans. Royal Soc. Lond. B326, 329–338.
4. R. C. Strohman (1997) Nature Biotechnol., 15, 194–200.
5. V. E. A. Russo, R. A. Martienssen, and A. D. Riggs, eds. (1996) Epigenetic Mechanisms of Gene Regulation, Cold Spring Harbor Laboratory Press, Cold Spring Harbor.
6. S. Loo and J. Rine (1995) Annu. Rev. Cell Dev. Biol. 11, 519–548.
7. S. G. Holmes, M. Braunstein, and J. R. Broach (1996) in Epigenetic Mechanisms of Gene Regulation, Cold Spring Harbor Laboratory Press, pp. 467–487.
8. B. T. Wakimoto (1998) Cell 93, 321–324.
9. V. Pirrotta (1998) Cell 93, 333–336.
10. M. A. Surani (1998) Cell 93, 309–312.
11. B. Panning and R. Jaenisch (1998) Cell 93, 305–308.
12. M. J. Singer and E. U. Selker (1995) In P. Meyer, ed., Gene Silencing in Higher Plants and Related Phenomena in Other Eukaryotes, pp. 165–177.
13. C. Goyon et al. (1994) J. Mol. Biol. 240, 42–51.
14. V. L. Chandler et al. (1996) In Epigenetic Mechanisms of Gene Regulation (V. E. A. Russo, R. A. Martienssen, and A. D. Riggs, eds.), Cold Spring Harbor Laboratory Press, Cold Spring Harbor, pp. 289–304.
الاكثر قراءة في مواضيع عامة في الاحياء الجزيئي
اخر الاخبار
اخبار العتبة العباسية المقدسة
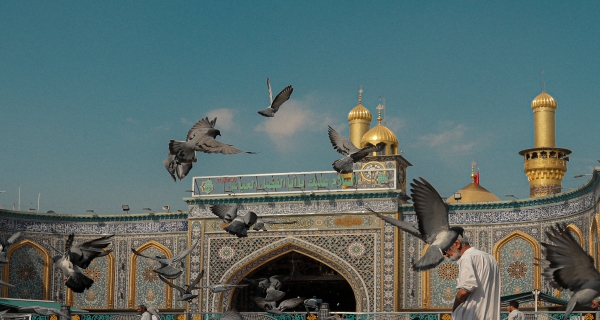
الآخبار الصحية
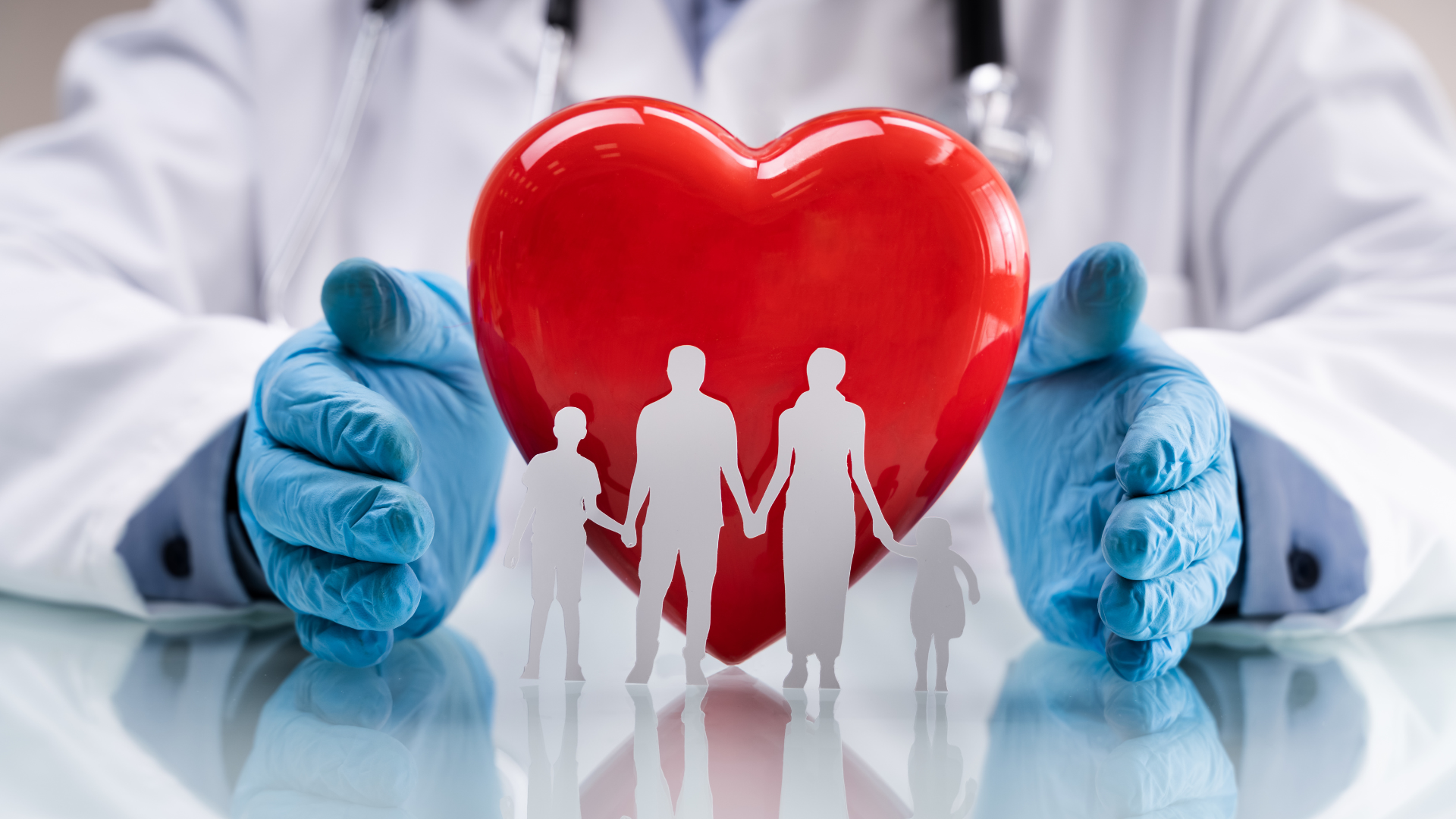