النبات
مواضيع عامة في علم النبات
الجذور - السيقان - الأوراق
النباتات الوعائية واللاوعائية
البذور (مغطاة البذور - عاريات البذور)
الطحالب
النباتات الطبية
الحيوان
مواضيع عامة في علم الحيوان
علم التشريح
التنوع الإحيائي
البايلوجيا الخلوية
الأحياء المجهرية
البكتيريا
الفطريات
الطفيليات
الفايروسات
علم الأمراض
الاورام
الامراض الوراثية
الامراض المناعية
الامراض المدارية
اضطرابات الدورة الدموية
مواضيع عامة في علم الامراض
الحشرات
التقانة الإحيائية
مواضيع عامة في التقانة الإحيائية
التقنية الحيوية المكروبية
التقنية الحيوية والميكروبات
الفعاليات الحيوية
وراثة الاحياء المجهرية
تصنيف الاحياء المجهرية
الاحياء المجهرية في الطبيعة
أيض الاجهاد
التقنية الحيوية والبيئة
التقنية الحيوية والطب
التقنية الحيوية والزراعة
التقنية الحيوية والصناعة
التقنية الحيوية والطاقة
البحار والطحالب الصغيرة
عزل البروتين
هندسة الجينات
التقنية الحياتية النانوية
مفاهيم التقنية الحيوية النانوية
التراكيب النانوية والمجاهر المستخدمة في رؤيتها
تصنيع وتخليق المواد النانوية
تطبيقات التقنية النانوية والحيوية النانوية
الرقائق والمتحسسات الحيوية
المصفوفات المجهرية وحاسوب الدنا
اللقاحات
البيئة والتلوث
علم الأجنة
اعضاء التكاثر وتشكل الاعراس
الاخصاب
التشطر
العصيبة وتشكل الجسيدات
تشكل اللواحق الجنينية
تكون المعيدة وظهور الطبقات الجنينية
مقدمة لعلم الاجنة
الأحياء الجزيئي
مواضيع عامة في الاحياء الجزيئي
علم وظائف الأعضاء
الغدد
مواضيع عامة في الغدد
الغدد الصم و هرموناتها
الجسم تحت السريري
الغدة النخامية
الغدة الكظرية
الغدة التناسلية
الغدة الدرقية والجار الدرقية
الغدة البنكرياسية
الغدة الصنوبرية
مواضيع عامة في علم وظائف الاعضاء
الخلية الحيوانية
الجهاز العصبي
أعضاء الحس
الجهاز العضلي
السوائل الجسمية
الجهاز الدوري والليمف
الجهاز التنفسي
الجهاز الهضمي
الجهاز البولي
المضادات الحيوية
مواضيع عامة في المضادات الحيوية
مضادات البكتيريا
مضادات الفطريات
مضادات الطفيليات
مضادات الفايروسات
علم الخلية
الوراثة
الأحياء العامة
المناعة
التحليلات المرضية
الكيمياء الحيوية
مواضيع متنوعة أخرى
الانزيمات
DNA Methylation
المؤلف:
M. Nelson, E. Raschke, and M. McClellend
المصدر:
Nucl. Acids Res.
الجزء والصفحة:
25-4-2016
3794
DNA Methylation
Enzymatic DNA methylation consists of the covalent attachment of a methyl group to a cytosine or adenosine residue in a defined sequence of double-strand DNA by a DNA methyltransferases. DNA methyltransferases use S-adenosyl-L-methionine (AdoMet or SAM) as the methyl-donating cofactor and are separated into two major classes. The first class methylates the C5 carbon of cytosine to form 5-methylcytosine (5mC). The second class methylates exocyclic amino nitrogen atoms to form either N-6-methyladenine (N6mA) or N-4-methylcytosine (N4mC). Eukaryotic DNA contains 5mC and prokaryotic DNA contains all three methylated bases: 5mC, N6mA, and N4mC. These methyl substituents are located in the major groove of DNA and disrupt interactions with some DNA-binding proteins and enzymes by perturbing binding or catalysis (1). These methyl-induced disturbances may be due to direct steric clashes combined with longer-range conformational changes produced in the DNA–protein interface (2) .
1. Prokaryotic Methylation
DNA methylation plays diverse roles in biology. For instance, methylation by the N-6-methyladenine DNA methyltransferase (Dam), found in Escherichia coli “marks” a DNA strand for repair by the methyl-directed mismatch repair system. In this repair system, a newly synthesized DNA strand, which lacks methyl groups, is scanned for mispairing of its bases with the original parental DNA strand that had been methylated by Dam MTase prior to replication. The parental strand serves as a template to direct the replacement of erroneously incorporated nucleotides. Shortly after proofreading and repair, Dam methylase modifies the new daughter strand to yield fully methylated DNA. In addition, Dam methylase plays an important role in DNA replication. High-efficiency E. coli chromosomal replication is dependent on eleven fully methylated Dam (GmATC( sites located at the oriC (the origin of replication). Although not lethal, loss of Dam methylation leads to increased spontaneous mutation rates and asynchronous DNA replication (3). Another prokaryotic N6mA methylase (CcrM) from Caulobacter crescentus is cell cycle–regulated and essential for viability. CcrM methylation at GATNC sites of daughter strands is temporally regulated at late stages of chromosomal replication and functions before cell division. Cells constitutively expressing CcrM throughout the cell cycle have abnormalities in their DNA replication and cell division. These findings indicate that the state of chromosomal DNA methylation regulates the progression of the cell cycle in this organism (4).
Prokaryotic methyltransferases, as part of restriction–modification systems, methylate host DNA sequences for protection against cleavage by the partner restriction enzyme. Methylation by enzymes like Dam that is involved in the normal functioning of host genomes may have been the evolutionary progenitors of restriction endonucleases and restriction–modification systems (5).
2. Eukaryotic Methylation
In eukaryotes, methylation functions in gene expression and regulation and cell division and development. Eukaryotic methylation is accomplished by DNA methyltransferases (DNA MTases( to form 5-methylcytosine residues at CG sites (6). Mutations that reduce cytosine methylation in fungi and plants result in abnormal chromosomal segregation and stability. Methylation may also serve to compartmentalize structurally large eukaryotic genomes (such as those of plants and vertebrates) into inactive, compact, methylated regions and unmethylated regions accessible to gene transcription and regulatory factors. In vertebrate genomes, 5-methylcytosine represents 1% of bases (7) . Vertebrate methylation is developmentally and tissue-specific and leads to defined, heritable patterns because of the preference of DNA MTase for hemimethylated DNA, specifically, DNA methylated on only one strand at a given methylation site (8). Li et al. (8) found that transfected mice embryos that were homozygous for a MTase mutation had 30% the normal amount of DNA methylation, major developmental abnormalities, and a recessive lethal phenotype. Methylation patterns have a role in genomic imprinting, where paternal and maternal alleles of a gene are differentially expressed. For instance, human and mouse insulin-like growth factor genes (Igf 2) are selectively expressed from the paternal gene, which, unlike the maternal allele, contains a methylated region upstream of the Igf 2 promoter. This finding suggests that the methylated region may interfere with the binding of a transcriptional repressor (9). Abnormal methylation of promoters of tumor suppressor genes promotes tumorigenesis (10). Interestingly, the spontaneous hydrolytic deamination of 5-methylcytosine residues in DNA produces thymidine; on replication, a transition mutation occurs that is responsible for one-third of human mutations (7).
3. Molecular Basis of Effects of Methylation
An understanding of the effects of methylation on local DNA structure, DNA-protein interactions, and catalysis provides insight into the multiple roles of methylation. For example, thermodynamic melting experiments using GATC-containing and methylated GmATC-containing decameric oligodeoxyribonucleotide duplexes show that the Tm value (the melting temperature at which half of the duplex population has dissociated into single strands) was 10° lower for the methylated oligomer, indicating that methylation had a destabilizing effect on the DNA (11). Studies were also conducted with EcoRI endonuclease using fully methylated oligomeric duplexes methylated at the second adenine containing the canonical sequence (GAmATTC) (the biologically relevant methylation site for EcoRI). Methylation destabilized the conformation of the DNA–protein interface, interrupting the precise structural geometry needed at the transition state for optimal catalysis and thereby prevented cleavage. Also, the cleavage rate constants for each strand on doubly methylated duplex were reduced ~600,000-fold compared to the unmethylated sequence. This rate reduction, combined with the lowered binding association rate caused by the methyl groups, explains why methylation effectively prevents EcoRI endonuclease cleavage (2).
DNA methylation is a ubiquitous, essential biological phenomenon in prokaryotes and eukaryotes. It is relevant to many areas of biology, molecular biology, biochemistry, and medicine. Examining DNA methylation at the molecular level also helps provide an understanding of the dynamic structure of DNA, protein–DNA interactions, and catalysis. Several uses for methylation of DNA in molecular cloning are discussed under Methyltransferase, DNA and Staggered Cut.
References
1. M. Nelson, E. Raschke, and M. McClellend (1993) Nucl. Acids Res. 21, 3139–3154.
2. L. Jen-Jacobsen, L. E. Engler, D. R. Lesser, M. R. Kurpiewski, C. Yee, and B. McVerry (1996) EMBO J. 15, 2870–2882.
3. B. R. Palmer and M. G. Marinus (1994) Gene 143, 1–12.
4. C. Stephens, A. Reisenauer, R. Wright, and L. Shapiro (1996) Proc. Natl. Acad. Sci. USA 93, 1210–1214.
5. T. A. Bickle and D. H. Kruger (1993) Microbiol. Rev. 57, 434–450.
6. W. C. Yen et al. (1992) Nucl. Acids Res. 20, 2287–2291.
7. T. H. Bestor and G. L. Verdine (1994) Curr. Opin. Cell Biol. 6, 380–389.
8. E. Li, T. H. Bestor, and R. Jaenisch (1992) Cell 69, 915–926.
9. A. Razin and H. Cedar (1994) Cell 77, 473–476.
10. G. L. Verdine (1994) Cell 76, 197–200
11. Q. Guo, M. Lu, and N. R. Kallenbach (1995) Biochemistry 34, 16359–16364.
الاكثر قراءة في مواضيع عامة في الاحياء الجزيئي
اخر الاخبار
اخبار العتبة العباسية المقدسة
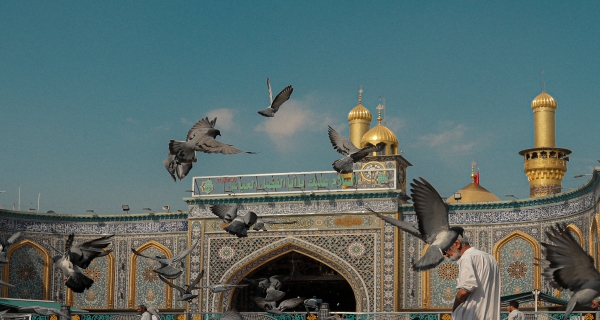
الآخبار الصحية
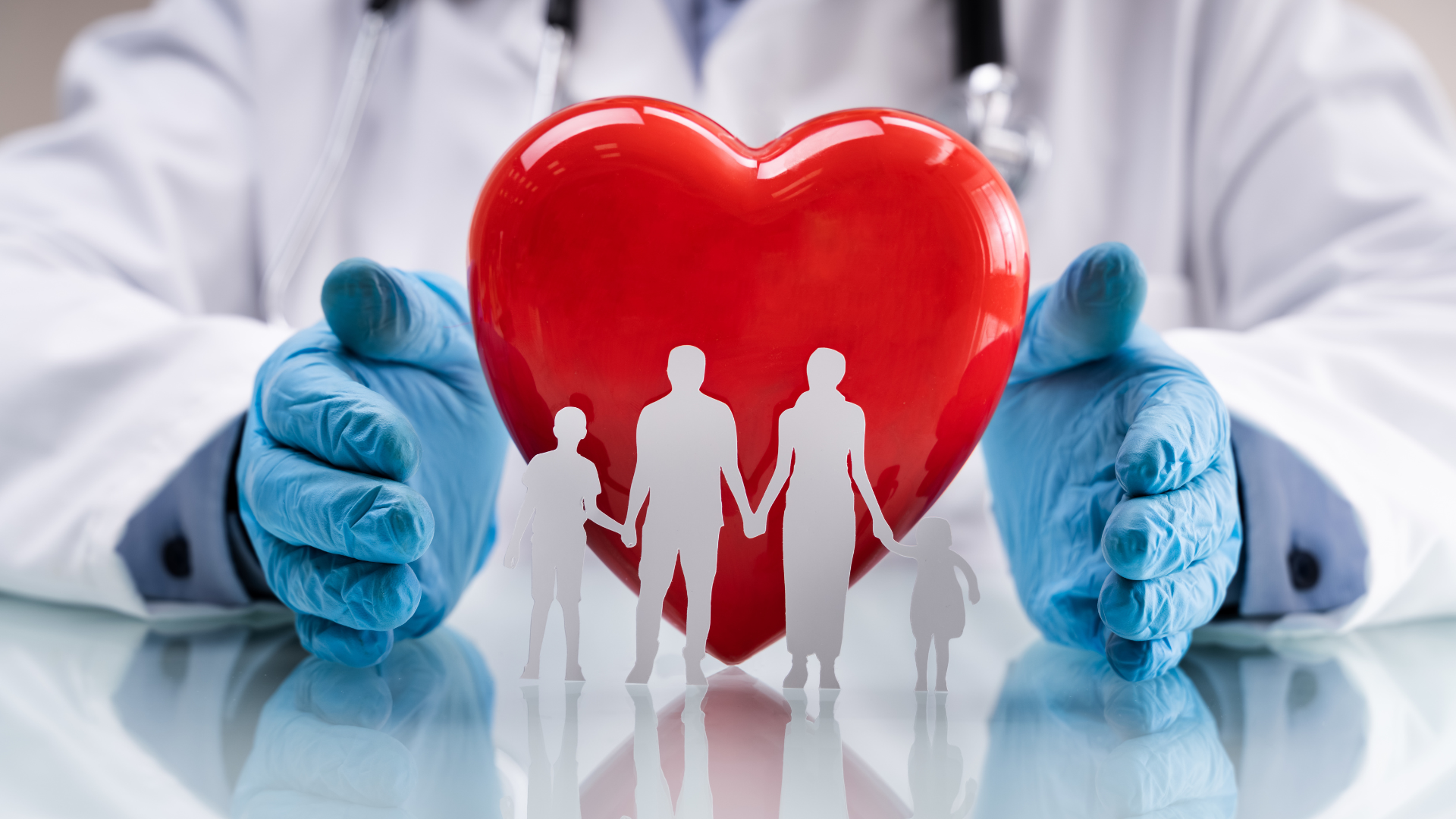