النبات
مواضيع عامة في علم النبات
الجذور - السيقان - الأوراق
النباتات الوعائية واللاوعائية
البذور (مغطاة البذور - عاريات البذور)
الطحالب
النباتات الطبية
الحيوان
مواضيع عامة في علم الحيوان
علم التشريح
التنوع الإحيائي
البايلوجيا الخلوية
الأحياء المجهرية
البكتيريا
الفطريات
الطفيليات
الفايروسات
علم الأمراض
الاورام
الامراض الوراثية
الامراض المناعية
الامراض المدارية
اضطرابات الدورة الدموية
مواضيع عامة في علم الامراض
الحشرات
التقانة الإحيائية
مواضيع عامة في التقانة الإحيائية
التقنية الحيوية المكروبية
التقنية الحيوية والميكروبات
الفعاليات الحيوية
وراثة الاحياء المجهرية
تصنيف الاحياء المجهرية
الاحياء المجهرية في الطبيعة
أيض الاجهاد
التقنية الحيوية والبيئة
التقنية الحيوية والطب
التقنية الحيوية والزراعة
التقنية الحيوية والصناعة
التقنية الحيوية والطاقة
البحار والطحالب الصغيرة
عزل البروتين
هندسة الجينات
التقنية الحياتية النانوية
مفاهيم التقنية الحيوية النانوية
التراكيب النانوية والمجاهر المستخدمة في رؤيتها
تصنيع وتخليق المواد النانوية
تطبيقات التقنية النانوية والحيوية النانوية
الرقائق والمتحسسات الحيوية
المصفوفات المجهرية وحاسوب الدنا
اللقاحات
البيئة والتلوث
علم الأجنة
اعضاء التكاثر وتشكل الاعراس
الاخصاب
التشطر
العصيبة وتشكل الجسيدات
تشكل اللواحق الجنينية
تكون المعيدة وظهور الطبقات الجنينية
مقدمة لعلم الاجنة
الأحياء الجزيئي
مواضيع عامة في الاحياء الجزيئي
علم وظائف الأعضاء
الغدد
مواضيع عامة في الغدد
الغدد الصم و هرموناتها
الجسم تحت السريري
الغدة النخامية
الغدة الكظرية
الغدة التناسلية
الغدة الدرقية والجار الدرقية
الغدة البنكرياسية
الغدة الصنوبرية
مواضيع عامة في علم وظائف الاعضاء
الخلية الحيوانية
الجهاز العصبي
أعضاء الحس
الجهاز العضلي
السوائل الجسمية
الجهاز الدوري والليمف
الجهاز التنفسي
الجهاز الهضمي
الجهاز البولي
المضادات الحيوية
مواضيع عامة في المضادات الحيوية
مضادات البكتيريا
مضادات الفطريات
مضادات الطفيليات
مضادات الفايروسات
علم الخلية
الوراثة
الأحياء العامة
المناعة
التحليلات المرضية
الكيمياء الحيوية
مواضيع متنوعة أخرى
الانزيمات
Principles of Electrophoresis
المؤلف:
Clive Dennison
المصدر:
A guide to protein isolation
الجزء والصفحة:
19-4-2016
2490
Principles of Electrophoresis
Active fractions isolated by a preparative fractionation procedure may be subjected to a number of analytical fractionation procedures to determine their purity. Analytical fractionations are distinguished from preparative fractionations by the criteria shown in Table 1.
In an analytical fractionation, therefore, a small amount of sample is sacrificed in order to gain information about the state of purity of the material being analyzed. Of the many physic-chemical techniques which have contributed to our knowledge of proteins (and nucleic acids), electrophoretic techniques occupy a position of primary importance. Electrophoresis finds its greatest usefulness in the analysis of mixtures and in the determination of purity, although certain forms of electrophoresis may be applied on a preparative scale.
Electrophoresis may be defined as the migration of charged ions in an electric field. In metal conductors, electric current is carried by the movement of electrons, largely along the surface of the metal. In solutions, the the “PA NIC” - Positive Anode Negative Cathode (NI) Not the Ions electric current flows between electrodes and is carried by ions. The negative electrode - the cathode - donates electrons and the positive electrode - the anode - takes up electrons to complete the circuit. The ions that result from the take up of electrons from the cathode will be negatively charged and will thus migrate towards the positive anode. Because of their anodic migration, negative ions are called “anions”.
Ions which result from the donation of an electron to the electron-deficient (i.e. positively charged) anode will themselves be electron deficient, and thus positively charged. These will migrate to the cathode and are thus called cations.
Figure 1. Electrophoresis: the movement of ions in an electric field.
There is a potential difference (voltage) between the anode and the cathode and if the solution between these is of constant composition and constant cross-section (i.e. constant resistance), the voltage gradient between them (dV/dx) will be linear, with units of volts cm-1. An ion placed in such an electric field will experience a force:-
Where,
F = electrophoretic force
K = a constant (embodying the Faraday constant and Avogadro's number
q = net charge on the protein (atomic charges/protein molecule)
This force will cause the protein to accelerate towards either the cathode or the anode, depending on the sign of its charge. As the protein moves it will experience a retarding frictional force (hydrodynamic drag), which at the speeds involved is proportional to the speed of movement.
Where,
It will be recalled that this situation is very similar to that obtaining during centrifugation , and the frictional coefficient can be determined in the same way.
Hence,
The proteins very soon reach terminal velocity, at which point the electrophoretic (propelling) force equals the frictional (retarding) force, i.e. from equations 1 and 2:
The free electrophoretic mobility, (µ), with units of (cm2 volt-1 sec-1) can be defined as the velocity per unit of voltage gradient, i.e.:
µ = velocity (voltage gradient)-1
Hence, from equation.3,
The electrophoretic mobility is thus a function of the charge on the protein ion and the medium through which it is travelling. Electrophoretic techniques exploit the fact that different ions have different mobilities in an electric field and so can be separated by electrophoresis.
The flow of electricity in electrophoresis is subject to the same physical laws as other forms of electricity. For example, Ohm's law applies:
Where
I = current (amps)
V = potential difference (volts)
R = resistance (ohms).
The unit of electrical charge is the coulomb and the unit of current [the ampere (amp)] may be defined as coulombs sec-1, i.e.,
The flow- of electricity involves work, which generates heat, and the work (W, in joules) done in transferring a charge of q coulombs between a potential difference of V volts is
And, since, from equ 5.,
Then,
From equ .7
Which means that I2Rt joules of heat will be developed in the conductor. The power (in watts) (defined as the rate of work) gives the rate of heating (joules sec-1).
Thus,
The effect of the buffer
The buffer in which electrophoresis is conducted, has a large influence on the migration of proteins. Firstly, the buffer pH will influence the charge (q) on the protein and hence the direction and speed of its migration. Secondly, the buffer ionic strength influences the proportion of the current carried by the proteins - at low ionic strength the proteins will carry a relatively large proportion of the current and so will have a relatively fast migration. At high ionic strength, most of the current will be carried by the buffer ions and so the proteins will migrate relatively slowly.
An analogy might be useful in visualizing this effect of ionic strength. Imagine a bank where there are two counters - one for deposits the anode) and one for withdrawals (= the cathode), with electrons being the money. The ions may be considered as customers waiting to be served at either counter, which one can visualize as being at opposite ends of the banking hall.
In Fig. 2, the circles represent customers queuing for service. In electrophoresis, these queues would be along the so-called field lines, which are usually (but not necessarily) straight lines. The lighter coloured circles represent buffer ion “customers” and the dark circles represent protein “customers”. When the “customer” at the counter is served, they move away, creating a “hole”. This “hole” is filled by the next customer in line, and so on, and so the “hole” moves backwards along the line. No matter how far away from a counter any customer is, they will be drawn towards the counter by the periodic appearance of a “hole” in the queue, immediately in front of them. If the counter assistants were very energetic (giving a high current) these “holes” would appear frequently and the customers would all progress quickly. On the other hand, if the counter assistants were lethargic (giving a low current) the “holes” would appear infrequently and progress of the customers would be slow.
Figure 2. A banking hall analogy of electrophoresis.
In Fig. 75, the relative proportions of protein ions to buffer ions shown is such that there is one protein ion in each queue. However, if we have the counter assistants working at the same rate (i.e. with the same current) but increase the number of customers (i.e. increase the ionic strength), then we will get the situation shown in Fig. 3.
With more buffer ions present, they will get most of the service (carry most of the current) and the progress of all ions in their respective queues, including the protein ions, will be slower.
In electrophoresis, therefore, a low ionic strength is preferred as it increases the rate of migration of proteins. A low ionic strength is also preferred as it gives a lower heat generation. Assuming a constant voltage, if the ionic strength is increased, the electrical resistance decreases but the current will increase. According to Eqn 8, heating is proportional to I2, but is only linearly affected by changes in resistance.
A high ionic strength buffer will therefore lead to greater heat generation, and so a low ionic strength is preferred.
Figure 3. Illustration of the effect of ionic strength in electrophoresis.
Strictly speaking, it is not the ionic strength per se which is the important factor in electrophoresis, but the mobility of the buffer ions. Thus at equivalent ionic strengths (i.e. at comparable buffering capacities), large buffer ions will migrate more slowly than small buffer ions (because of their greater frictional coefficient, f). Large buffer ions will thus lead to less heat generation and a faster migration of the proteins. For example, barbitone (I) has a mobility about one quarter of that of Tris (II), and can therefore be used at four times the concentration of Tris - at which concentration it will be roughly four times more effective as a buffer.
The effect of ionic strength is actually more complex than indicated in the simplistic model given above. The reason is that ionic strength also has an effect on the electrical double layer which surrounds proteins in solution. The ions in the electrical double layer have the effect of decreasing the apparent charge on the protein. As the protein moves under electrophoresis, it takes with it a part of the electrical double layer. As the ionic strength increases, the thickness of the electrical double layer decreases and more of the counterions are drawn along with the migrating protein, effectively reducing its charge. The mobility of the protein thus decreases with increasing ionic strength. A more complete discussion of this effect is given by Kyte .
References
Dennison, C. (2002). A guide to protein isolation . School of Molecular mid Cellular Biosciences, University of Natal . Kluwer Academic Publishers new york, Boston, Dordrecht, London, Moscow .
الاكثر قراءة في عزل البروتين
اخر الاخبار
اخبار العتبة العباسية المقدسة
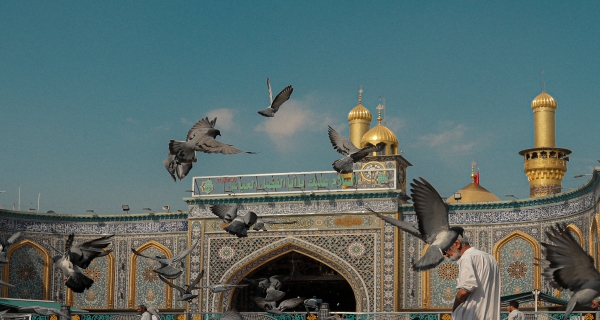
الآخبار الصحية
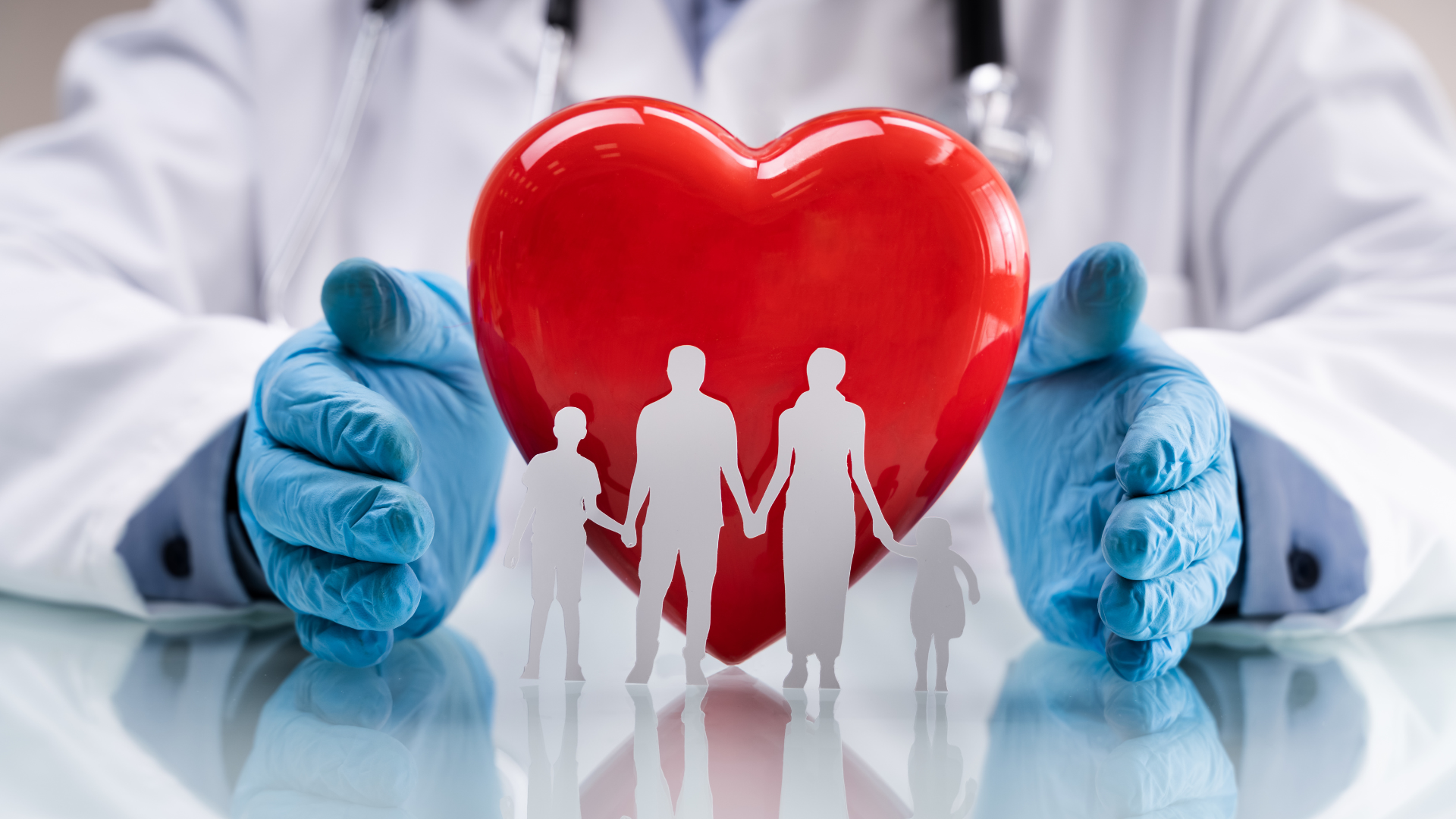