تاريخ الفيزياء
علماء الفيزياء
الفيزياء الكلاسيكية
الميكانيك
الديناميكا الحرارية
الكهربائية والمغناطيسية
الكهربائية
المغناطيسية
الكهرومغناطيسية
علم البصريات
تاريخ علم البصريات
الضوء
مواضيع عامة في علم البصريات
الصوت
الفيزياء الحديثة
النظرية النسبية
النظرية النسبية الخاصة
النظرية النسبية العامة
مواضيع عامة في النظرية النسبية
ميكانيكا الكم
الفيزياء الذرية
الفيزياء الجزيئية
الفيزياء النووية
مواضيع عامة في الفيزياء النووية
النشاط الاشعاعي
فيزياء الحالة الصلبة
الموصلات
أشباه الموصلات
العوازل
مواضيع عامة في الفيزياء الصلبة
فيزياء الجوامد
الليزر
أنواع الليزر
بعض تطبيقات الليزر
مواضيع عامة في الليزر
علم الفلك
تاريخ وعلماء علم الفلك
الثقوب السوداء
المجموعة الشمسية
الشمس
كوكب عطارد
كوكب الزهرة
كوكب الأرض
كوكب المريخ
كوكب المشتري
كوكب زحل
كوكب أورانوس
كوكب نبتون
كوكب بلوتو
القمر
كواكب ومواضيع اخرى
مواضيع عامة في علم الفلك
النجوم
البلازما
الألكترونيات
خواص المادة
الطاقة البديلة
الطاقة الشمسية
مواضيع عامة في الطاقة البديلة
المد والجزر
فيزياء الجسيمات
الفيزياء والعلوم الأخرى
الفيزياء الكيميائية
الفيزياء الرياضية
الفيزياء الحيوية
الفيزياء العامة
مواضيع عامة في الفيزياء
تجارب فيزيائية
مصطلحات وتعاريف فيزيائية
وحدات القياس الفيزيائية
طرائف الفيزياء
مواضيع اخرى
THREE AND FOUR-LEVEL LASERS
المؤلف:
Mark Csele
المصدر:
FUNDAMENTALS OF LIGHT SOURCES AND LASERS
الجزء والصفحة:
p119
16-3-2016
9520
THREE AND FOUR-LEVEL LASERS
Lasers are classed by the number of energy levels involved in the actual lasing process as three or four-level lasers. In a three-level system (the simplest), energy injected into the gain medium excites atoms to a pump level above the upper lasing level. From there atoms decay to the upper lasing level. This decay to the upper lasing level usually occurs by emitting heat, not photons. It is rapid and quickly populates the upper energy level. This upper level often has a long lifetime, so a healthy population of atoms builds in that level. Lasing transitions now occur between the upper level and the ground state, emitting laser light in the process. This system is characterized by the lack of a discrete lower lasing level; the ground state serves that purpose. The left side of Figure 1.1 shows the energies involved in a three level laser, including the pump, upper lasing level, and lower-lasing levels.
Four-level systems feature a discrete lower lasing level between the upper and ground states. Atoms making a laser transition to the lower state decay further to the ground state, in some cases by emitting a photon (in the case of an argon-ion laser, a 74-nm photon is emitted during this decay to the ground state). Four-level lasers are by far the most common. In a helium–neon laser, for example, the pump level is in helium (it need not be in the same species as the lasing atom), while the upper and lower lasing levels are in neon. A carbon dioxide laser has a similar situation, where the pump level is in nitrogen gas, which like helium in a
Figure 1.1. Ideal three and four-level lasers.
helium–neon laser, is in larger proportions than the lasing species. It is also possible in some lasers, such as metal-vapor lasers, to pump directly to the upper lasing level (i.e., there is no pump level). In this case, atoms are excited to the upper lasing level, where they make a transition to the lower lasing level, finally decaying to the ground state. These are particularly efficient systems since they lack an energy loss when atoms in the pump level decay to the upper lasing level (which, in most cases, shows up as heat in the laser). Although the metal-vapor system has only three levels, it has more in common with a four-level system than does a classic three level system.
In a four-level laser, laser gain is realized as soon as pump energy is applied to the system. Pump energy is injected into the pump level, where it decays, in most cases almost instantaneously, to the upper lasing level. Assuming that the upper level has a longer lifetime than the lower level (and in most four-level lasers it does), a population inversion occurs almost immediately after pump energy is injected. Although there may not be a usable output beam (since any small gain produced will be lost to absorption and other losses within the system until threshold is reached), there is a population inversion and hence a gain. Injecting a little more energy into the system will raise the gain to a level where it exceeds lasing threshold and a usable output beam appears.
So the nature of a four-level laser is such that a population inversion is easy to achieve but what happens in a three-level laser where the lower lasing level is the ground level? We know from Boltzmann statistics that at a given operating temperature there will be an enormous population of lasing atoms at ground state, and that to achieve a laser gain we must raise over half of these to the upper lasing level, which in many cases is far above ground. Consider the dynamics of the ruby laser system in Figure 1.2, in which the upper lasing level is about 1.8 eV above ground. The thermal population of this level at 300 K is negligible. Also evident in the figure are two pump bands in the blue and green regions of the spectrum.
Strong pumping is required for such a laser to achieve a population inversion, and these lasers exhibit a high threshold of pump energy. A considerable amount of pump energy is required just to achieve inversion, let alone lasing threshold! There is also a time delay between the onset of pumping power and lasing output. As pump energy is injected into the ruby, one must wait until a significant population of atoms (certainly, over half of the chromium ions in the rod) reaches the upper lasing level before lasing can begin. So why does ruby work quite well as a laser material (after all, it was the first)? The reason lies in the two broad pump bands, which readily absorb energy from a flash lamp (xenon flash lamps, you will recall from an earlier discussion, emit a good portion of their radiation in the violet to green region, so are commonly used with ruby lasers). As well as being broad, the pump bands have incredibly short lifetimes (on the order of 1 μs), which causes energy absorbed into these pump bands to relax almost immediately to the upper lasing level. Finally, the lifetime of the upper level, 3 ms, is quite long, allowing the excited ions to remain there long enough to have a good chance of emitting light by stimulated (as opposed to spontaneous) emission. Although most ruby lasers are pulsed, it is possible to operate the
Figure 1.2. Dynamics of the ruby laser.
material as a CW laser. Special conditions are required, however, such as extreme cooling to depopulate a portion of the ground level; this is, not surprisingly, a collection of closely spaced levels. Doing so allows the system to operate more like a four-level system, as described next.
Now, in contrast to the three-level ruby laser, consider a four-level laser such as the YAG (also a solid-state laser), with energy levels as depicted in Figure 1.3. The YAG system is characterized by a cluster of pump levels from which excited atoms decay rapidly to the upper laser level. There are multiple pump levels, allowing the
Figure 1.3. Energy levels in the YAG laser.
system to absorb energy at a variety of wavelengths, including the important band at 790 to 810 nm, useful for pumping via a semiconductor laser. All pump levels have short lifetimes, around 100 ns, and decay rapidly to the upper lasing level. The upper level has a very long lifetime of 1.2 ms compared to the lower level, which decays to ground in 30 ns. Many lasing transitions are possible in this system, including the most powerful, at 1064 nm. The lower lasing level of the transition at 946 nm is extremely close to the ion ground state. Because of the large thermal populations of the lower lasing level, lasing on this transition occurs in a mode resembling a three-level laser, and strong pumping is required to allow this transition to oscillate. For other transitions such as 1064 nm, the situation is more favorable and a population inversion will occur quickly.
Most lasers are four-level lasers: for example, the familiar He Ne laser with energy levels pictured in Figure 1.4. The pumping mechanism, involving resonant energy levels between helium and neon, has been described previously, but an examination of the lower levels (of which there are many) reveals an intervening metastable state (so-called because it has a relatively long lifetime, making it almost stable) to which atoms in one of the lower lasing levels can rapidly decay. This pathway allows depopulation of the lower lasing levels, making population inversion easy to achieve. This rapid decay is radiative; it is accompanied by spontaneous emission of light at about 600 nm. This light does not contribute to laser action but is seen as an orange line in the spectrum of the tube emission as viewed through a spectroscope. It is part of the pink glow coming from the length of the tube. Not
Figure 1.4. Helium–neon laser energy levels.
surprisingly, atoms at the lower level must emit the energy somewhere when making the transition to a yet lower energy level, and the 600-nm spontaneous emission provides that outlet. From that metastable state they must collide with the walls of the tube to return to ground state.
This scenario illustrates that the He Ne laser involves four energy levels in the active lasing atom itself (i.e., the neon atom) plus a pump level in helium. The neon levels are the ground state, the upper lasing level, the lower lasing level, and the metastable state, from which it returns to ground state. Although it is tempting to call it a five-level laser, it is classed as a four-level system since only four states are really involved with the laser process. Other levels are simply there as stepping stones. The process begins again when ground-state neon atoms are again pumped by collision with energetic helium atoms. Some lasers feature hybrid-level systems. One example is the copper-vapor laser, which has a three level system which more closely resembles a four-level system since it lacks a pump level (Figure 1.5). In this system, energy is injected directly into the upper lasing level (ULL) (by direct collision of electrons with copper atoms), but unlike a true three-level system, it does have a discrete lower lasing level (LLL).
Although a system such as this could theoretically offer high efficiencies since no energy is wasted in the decay of energy from the pump level to the upper lasing level, this particular laser does not operate in CW mode, due to an unfavorable situation in which the lifetime of the ULL is shorter than that of the LLL.
Perhaps the most dramatic effect of the type of energy-level system is the amount of pump energy required to generate laser action. In any laser, output can begin as soon as population inversion occurs, followed by stimulated emission. Gain increases until it exceeds total losses in the system and laser output occurs. In a three-level laser it takes a large amount of pump energy just to generate an inversion, since the population of the ULL must exceed that of the ground state. In a four-level
Figure 1.5. Copper-vapor laser energy levels.
laser, inversion can be achieved with much lower pump energies since the LLL is a discrete level that is not populated to nearly the level of the ground state. Assuming that the LLL for a four-level laser is a few electron volts above ground state, it will not be thermally populated to any large extent unlike the three-level laser, in which the ground state has a large population, making inversion difficult to achieve.
الاكثر قراءة في مواضيع عامة في الليزر
اخر الاخبار
اخبار العتبة العباسية المقدسة
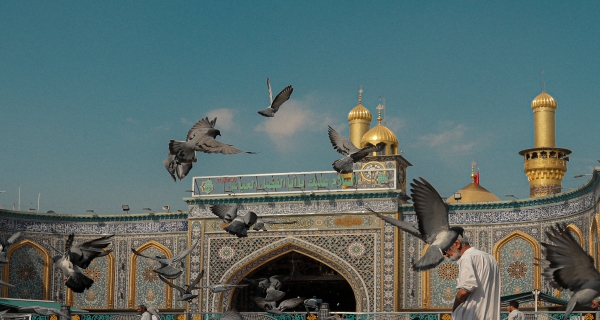
الآخبار الصحية
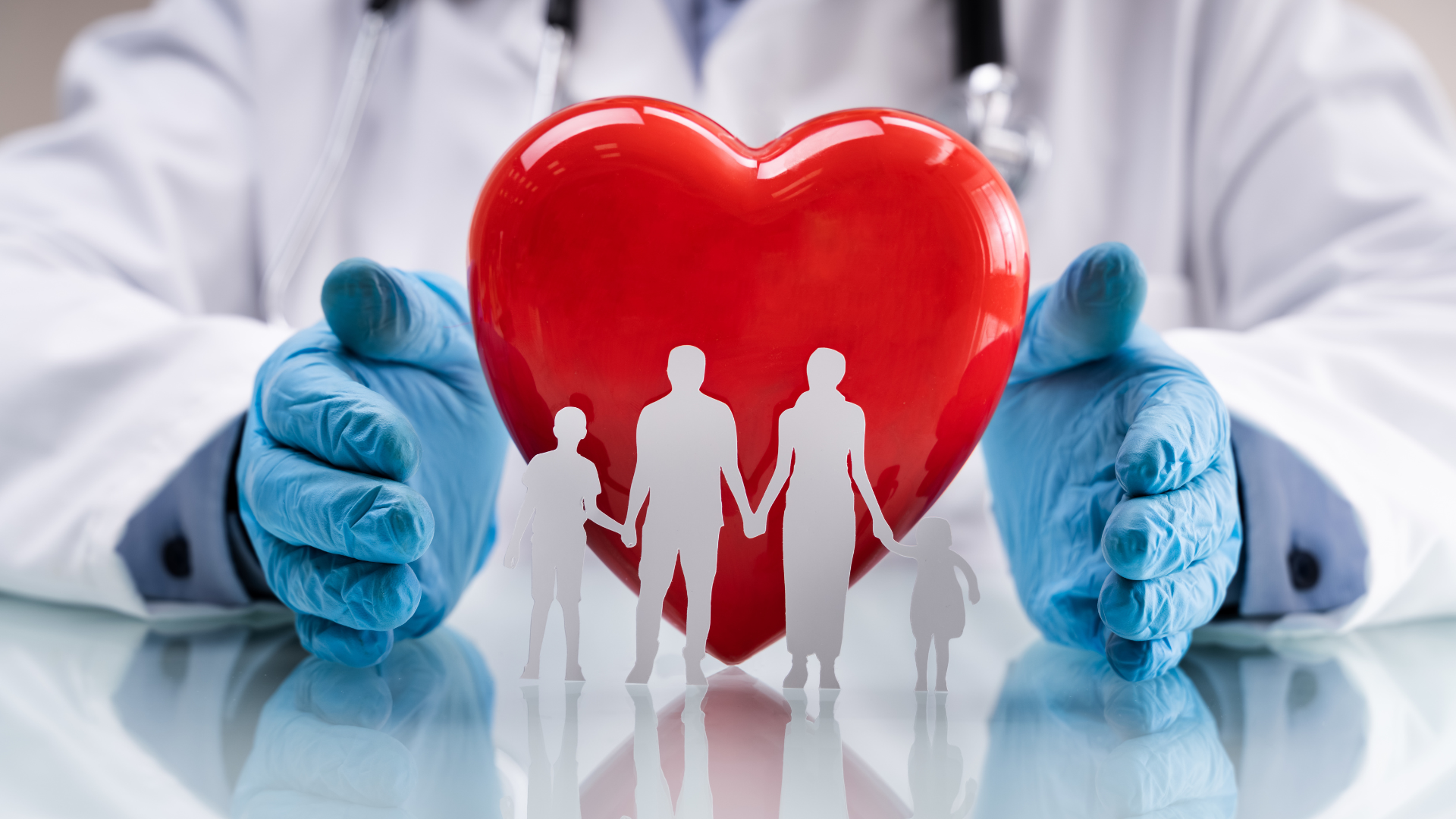