النبات
مواضيع عامة في علم النبات
الجذور - السيقان - الأوراق
النباتات الوعائية واللاوعائية
البذور (مغطاة البذور - عاريات البذور)
الطحالب
النباتات الطبية
الحيوان
مواضيع عامة في علم الحيوان
علم التشريح
التنوع الإحيائي
البايلوجيا الخلوية
الأحياء المجهرية
البكتيريا
الفطريات
الطفيليات
الفايروسات
علم الأمراض
الاورام
الامراض الوراثية
الامراض المناعية
الامراض المدارية
اضطرابات الدورة الدموية
مواضيع عامة في علم الامراض
الحشرات
التقانة الإحيائية
مواضيع عامة في التقانة الإحيائية
التقنية الحيوية المكروبية
التقنية الحيوية والميكروبات
الفعاليات الحيوية
وراثة الاحياء المجهرية
تصنيف الاحياء المجهرية
الاحياء المجهرية في الطبيعة
أيض الاجهاد
التقنية الحيوية والبيئة
التقنية الحيوية والطب
التقنية الحيوية والزراعة
التقنية الحيوية والصناعة
التقنية الحيوية والطاقة
البحار والطحالب الصغيرة
عزل البروتين
هندسة الجينات
التقنية الحياتية النانوية
مفاهيم التقنية الحيوية النانوية
التراكيب النانوية والمجاهر المستخدمة في رؤيتها
تصنيع وتخليق المواد النانوية
تطبيقات التقنية النانوية والحيوية النانوية
الرقائق والمتحسسات الحيوية
المصفوفات المجهرية وحاسوب الدنا
اللقاحات
البيئة والتلوث
علم الأجنة
اعضاء التكاثر وتشكل الاعراس
الاخصاب
التشطر
العصيبة وتشكل الجسيدات
تشكل اللواحق الجنينية
تكون المعيدة وظهور الطبقات الجنينية
مقدمة لعلم الاجنة
الأحياء الجزيئي
مواضيع عامة في الاحياء الجزيئي
علم وظائف الأعضاء
الغدد
مواضيع عامة في الغدد
الغدد الصم و هرموناتها
الجسم تحت السريري
الغدة النخامية
الغدة الكظرية
الغدة التناسلية
الغدة الدرقية والجار الدرقية
الغدة البنكرياسية
الغدة الصنوبرية
مواضيع عامة في علم وظائف الاعضاء
الخلية الحيوانية
الجهاز العصبي
أعضاء الحس
الجهاز العضلي
السوائل الجسمية
الجهاز الدوري والليمف
الجهاز التنفسي
الجهاز الهضمي
الجهاز البولي
المضادات الحيوية
مواضيع عامة في المضادات الحيوية
مضادات البكتيريا
مضادات الفطريات
مضادات الطفيليات
مضادات الفايروسات
علم الخلية
الوراثة
الأحياء العامة
المناعة
التحليلات المرضية
الكيمياء الحيوية
مواضيع متنوعة أخرى
الانزيمات
Blood Clotting
المؤلف:
Butenas and K. G. Mann
المصدر:
Biochemistry 35, 1904–1910
الجزء والصفحة:
14-12-2015
3966
Blood Clotting
The clotting or coagulation of blood is necessary for the maintenance of vascular integrity, and is therefore essential to survival. Cessation of bleeding can be achieved within minutes, involving both cellular and soluble protein components present in blood. The overall function of the blood coagulation system is the rapid amplification of a small initial stimulus via a series of sequential reactions that activate various zymogens and pro-cofactors of serine proteinases, culminating in the generation of thrombin, which proteolytically converts fibrinogen to fibrin. Fibrin monomers subsequently polymerize to form the insoluble fibrin clot. The major enzymatic components of the coagulation pathways are a series of homologous serine proteinases whose function is determined primarily by differences in the organization of their N terminal, noncatalytic domains. Most of the reactions are catalyzed by complexes between these serine proteinases, nonenzymatic protein cofactors, and serine proteinase zymogens that are assembled on phospholipid surfaces in the presence of calcium ions. These complexes are able to catalyze activation of the proteinase zymogen by limited proteolysis at rates up to 106-fold greater than those in the absence of cofactor/phospholipid. The proteinase product of one reaction then participates in another analogous complex, thus providing the amplification, as well as the multiple levels at which both positive and negative regulation can be exerted.
Figure 1 shows the two classical pathways of blood coagulation, termed the intrinsic and extrinsic pathways. These were originally envisaged as elegant, essentially linear sequences of reactions, but feedback inhibition reactions and cross-reactions between the two pathways are now known to be important and to provide further amplification. The terminology used to describe the two pathways of blood coagulation is somewhat counterintuitive, tending to imply the opposite to what is now known to be the physiological clotting mechanism. Genetic deficiencies in the extrinsic pathway lead to severe bleeding tendencies, but initiation of this pathway in vitro requires components in the form of tissue extracts (containing tissue factor and phospholipid) that are not present in blood. By contrast, the physiological role of the “intrinsic” pathway is unclear, as genetic deficiencies in its components do not give rise to bleeding disorders, but all the components are present in blood, and it can be initiated by simply removing blood from the circulation. This is due to contact with a negatively charged surface, such as glass, giving the pathway its alternative name of “contact activation,” and involves proteinases and cofactors quite distinct from those of the extrinsic pathway.
Figure 1. The proteolytic reactions of the blood clotting pathways. The zymogen/proteinase pairs are shown in black with the “forward” reactions as solid lines, the cofactors are shown in blue with the positive feedback reactions as dashed blue lines. The proteolytic negative feedback reactions are shown in red. The complexes initiating the two pathways of coagulation are boxed: the factor VII–tissue factor complex and the contact activation complex that assembles on high molecular-weight kininogen (HMWK). The Roman numeral system used for the coagulation proteinases and cofactors is based loosely on the order in which they were discovered, with the exceptions of the first four components fibrinogen, prothrombin, tissue factor–phospholipid complex, and Ca2+. The proteolytically activated forms of these factors are denoted by a subscript “a,” for example, factor Xa. Additional nonproteolytic inhibitory mechanisms exist, the principal of which are the serpin antithrombin (an inhibitor of most of the Gla-proteinases) and tissue factor pathway inhibitor (TFPI), a Kunitz-type proteinase inhibitor of factors VIIa and Xa.
A complete description of the coagulation system and the many proteolytic and inhibitory reactions that lead to its exquisite functional regulation is beyond the scope of this article. We will therefore focus on two key proteolytic steps that are representative of the two types of multicomponent complexes of the extrinsic pathway and that demonstrate the fundamental interactions and mechanisms underlying catalysis of the proteolytic reactions of blood coagulation; one requiring proteolytically activated soluble cofactors, the other requiring integral membrane protein cofactors that do not require proteolytic activation.
1.Regulation of Blood Clotting by Complexes Involving Soluble Protein Cofactors
The only proteolytic reaction common to both pathways is the activation of prothrombin, the final serine proteinase zymogen of the coagulation cascade. Factor Xa (i.e., activated factor X) is the proteinase component of the “prothrombinase complex” that catalyzes the generation of thrombin. Both zymogen and active proteinase have an N-terminal “Gla domain,” which contains g-carboxyglutamic acid residues. The Gla domain is also present in prothrombin (as well as factors VII and IX, and protein C, the so-called vitamin K–dependent or Gla proteinases); it binds Ca2+ and participates in binding to negatively charged phospholipid surfaces, which in vivo are provided
principally by activated platelets. Therefore, both enzyme and substrate bind to phospholipid surfaces, with dissociation constants of 0.1–1 µM (1). The cofactor of the prothrombinase complex is factor Va, which is generated by thrombin-catalyzed limited proteolysis of factor V, providing one of the major feedback activation reactions. Factor Va also binds to negatively-charged phospholipid surfaces, but with a much lower dissociation constant of ~ 5 nM (2). It does not contain a Gla domain, and binds through a different mechanism involving partial insertion of the protein into the lipid bilayer; it is often viewed as a membrane binding site for factor Xa. The latter thus incorporates into the complex by both protein–phospholipid and protein–protein interactions. Kinetic experiments suggest that the two phospholipid-bound components associate by lateral diffusion, but the interactions involved are complex and to some extent cooperative. Although prothrombin also binds to phospholipid, it is not clear whether the relevant substrate is phospholipid-bound prothrombin or prothrombin in the solution phase but at a high local concentration in the vicinity of the membrane surface, which can be several orders of magnitude above that in bulk solution. The kinetic effect of prothrombin binding to phospholipid is a reduction in the apparent Km (Michaelis constant) for prothrombin activation (“apparent” as the Km does not reflect the true concentration at the phospholipid–surface interface). This is of physiological significance, since the Km falls from ~ 100 µM to < 1 µM (3), compared to a concentration of 1.4 µM in blood plasma. In addition to this effect, the catalytic rate constant, kcat, for prothrombin activation is greatly increased, primarily as a consequence of the Xa–Va interaction (Table 1). This suggests that the active-site environment of the proteinase is significantly affected by complex formation, although the molecular basis of this effect is not known. The prothrombinase complex also helps to achieve maximum catalytic advantage by ordering the sequence of the two proteolytic cleavages required for prothrombin activation.
Table 1. Effect of Various Components of Prothrombinase Complex on Kinetic Parameters for Prothrombin Activation0
The phospholipid-bound complex of factor IXa (proteinase) and factor VIIIa (cofactor) that activates factor X has molecular and functional characteristics very similar to those of the prothrombinase complex. Factor VIII shares homology with factor V and is also activated by thrombin; factor IX has the same domain structure as factor X and is also activated by factor VIIa. There is evidence that the phospholipid-binding properties of these proteinase–zymogen components enable the proteinase product of one complex to be transferred to the subsequent complex as its enzyme without dissociation from the membrane, thereby giving a further catalytic advantage (4).
2.Regulation of Blood Clotting by Complexes Involving Integral Membrane Proteins Two integral membrane proteins with quite distinct and contrasting functions are involved in the regulation of blood coagulation: tissue factor and thrombomodulin, which are involved, respectively, in the initiation and termination of coagulation. Blood coagulation subsequent to injury of the vasculature is initiated by the exposure of tissue factor, a nonenzymatic cofactor widely expressed by cells of the subendothelium of blood vessels (although its expression can also be induced in endothelial cells and monocytes). Tissue factor binds factor VIIa, a Gla-proteinase, with high affinity (Kd = 1–5 nM) (5). The structure of this complex has been determined by X-ray crystallography (6;( the extracellular part of tissue factor is composed of two homologous C2-type immunoglobulin-like modules, and the protein is most closely related to the IFNg receptor, a member of the class 2 cytokine receptors (see Interferons). Factor VIIa is engaged in multiple contacts with both of the tissue factor modules.
The classical substrate for factor VIIa in this complex is factor X, but it is now known that factor IX is also a physiologically relevant substrate (7). Activation of these substrates, and the subsequent incorporation of their activated forms into their cognate complexes, thus propagates the initial stimulus proteolytically. In contrast to factors V and VIII, tissue factor is constitutively active as a cofactor and does not require proteolytic processing. However, factor VII does need proteolytic activation for the complex to be active, and what initially catalyzes this cleavage and thus constitutes the initial proteolytic event in the coagulation cascade has not been a trivial problem to address, because of the extraordinary sensitivity of the system to proteolytic activation, and it remains a topic of controversy. Proposals have included (1) that factor VII is not a true zymogen and possesses a significant degree of intrinsic proteolytic activity, (2) induction of activity in factor VII by tissue factor, (3) autoactivation of factor VII, (4) and activation of factor VII by trace amounts of activated proteinases. Although not demonstrated unambiguously, mechanism 4 appears to be favored, as factor VII can be activated by factors VIIa, IXa, Xa, and thrombin in vitro. These may be generated by the contact activation system, alternative mechanisms, or “leakage” of the system, but in such low amounts that they are insignificant in the absence of tissue factor. The principal activator of factor VII once the system is initiated is considered to be factor Xa (8). Tissue factor has little or no effect on factor VII activation, but assembly of the tissue factor complex increases factors IX and X activation by up to 104-fold (9). Both the kinetic mechanisms and the molecular interactions responsible for this effect are analogous to those described for the prothrombinase complex, which involves the optimal presentation of the zymogen substrate to the catalytically efficient proteinase:cofactor complex for maximum catalytic advantage.
The other integral membrane protein of the coagulation system, thrombomodulin, is expressed by endothelial cells and acts as a cofactor for the proteolytic activation of the negative regulator of the coagulation system, protein C (10). Activated protein C (a Gla-proteinase) proteolytically inactivates the procoagulant cofactors factors Va and VIIIa. In common with the principal feedback activation reactions, thrombin is again involved. But in this case, rather than proteolytically activating the cofactor, it is the enzymatic component of the thrombomodulin complex; thus thrombin can fully modulate its own generation. The interaction with thrombomodulin dramatically alters the substrate specificity of thrombin, such that protein C activation is increased 104-fold, while its activity toward factor V and fibrinogen is diminished (1). Although protein C can bind phospholipid through its Gla domain, this interaction plays only a minor part in these effects and, because thrombin lacks a Gla domain, protein–protein interactions are the major determinants in thrombomodulin complex anticoagulant function, in contrast to the procoagulant complexes described above.
References
1.C. T. Esmon, N. L. Esmon, and K. W. Harris (1982) J. Biol. Chem. 257, 7944–7947.
2.S. Krishnaswamy and K. G. Mann (1988) J. Biol. Chem. 263, 5714–5720.
3.J. Rosing, G. Tans, J. W. Govers-Riemslag, R. F. Zwaal, and H. C. Hemker (1980) J. Biol. Chem. 255, 274–283.
4.K. G. Mann, M. E. Nesheim, W. R. Church, P. Haley, and S. Krishnaswamy (1990) Blood 76, 1-16.
5.R. F. Kelley, K. E. Costas, M. P. O''Connell, and R. A. Lazarus (1995) Biochemistry 34, 10383–10392.
6.D. W. Banner, A. D''Arcy, C. Chene, F. K. Winkler, A. Guha, W. H. Konigsberg, Y. Nemerson, and D. Kirchhofer (1996) Nature, 380, 41–46.
7.K. A. Bauer, P. M. Mannucci, A. Gringeri, F. Tradati, S. Barzegar, B. L. Kass, H. ten Cate, A. S. Kestin, D. B. Brettler, and R. D. Rosenberg (1992) Blood 79, 2039–2047.
8.S. Butenas and K. G. Mann, (1996) Biochemistry 35, 1904–1910.
9. M. Zur and Y. Nemerson (1980) J. Biol. Chem. 255, 5703–5711.
10. C. T. Esmon (1995) FASEB J. 9, 946–955.
الاكثر قراءة في مواضيع عامة في الاحياء الجزيئي
اخر الاخبار
اخبار العتبة العباسية المقدسة
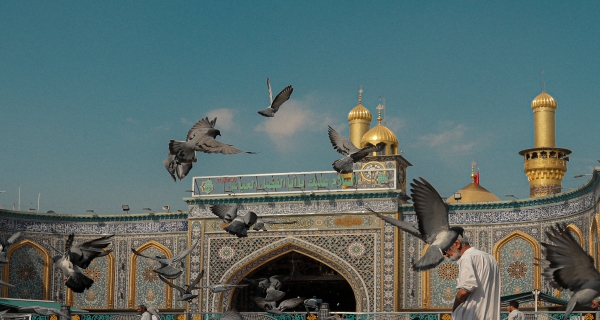
الآخبار الصحية
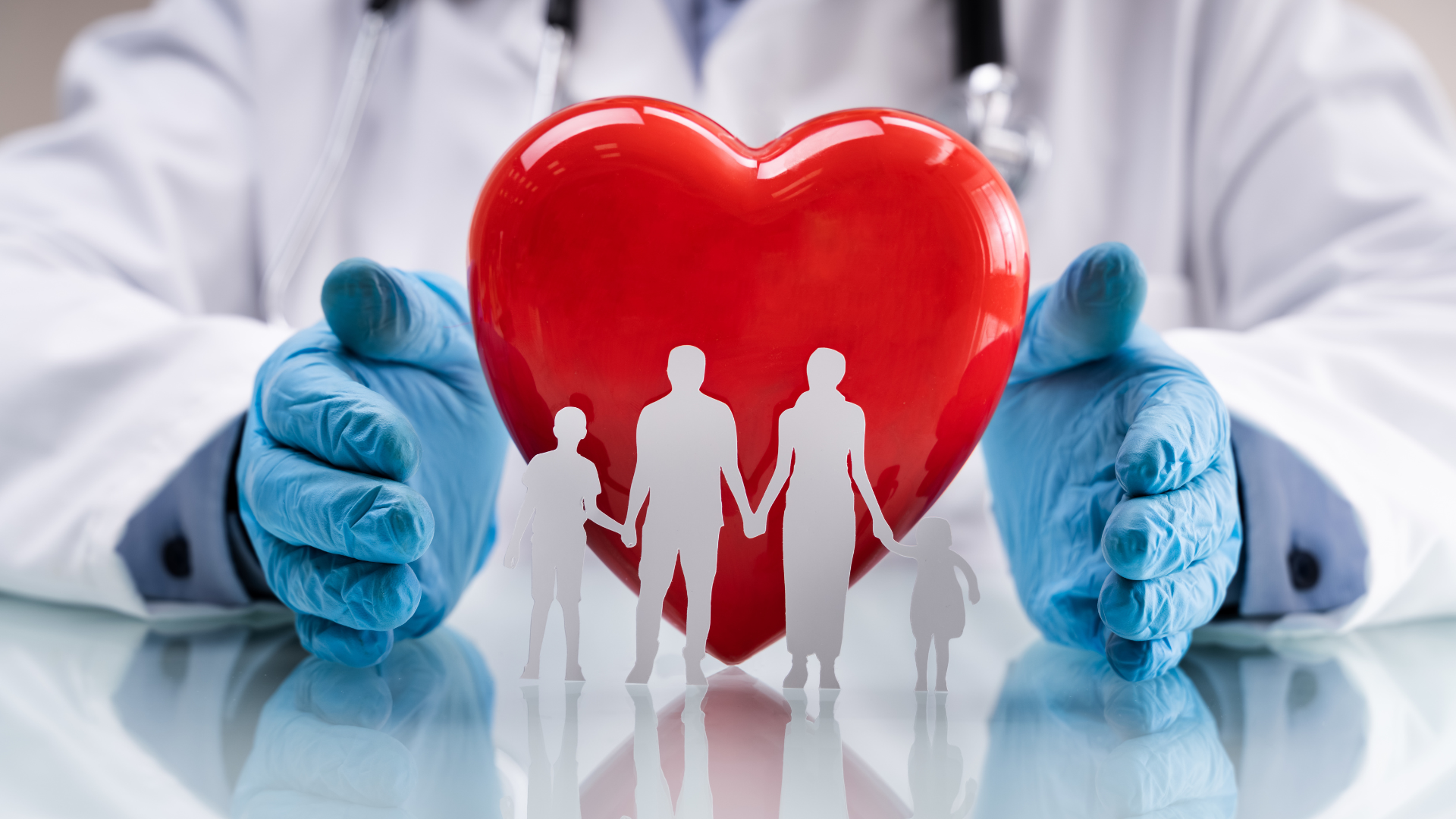