النبات
مواضيع عامة في علم النبات
الجذور - السيقان - الأوراق
النباتات الوعائية واللاوعائية
البذور (مغطاة البذور - عاريات البذور)
الطحالب
النباتات الطبية
الحيوان
مواضيع عامة في علم الحيوان
علم التشريح
التنوع الإحيائي
البايلوجيا الخلوية
الأحياء المجهرية
البكتيريا
الفطريات
الطفيليات
الفايروسات
علم الأمراض
الاورام
الامراض الوراثية
الامراض المناعية
الامراض المدارية
اضطرابات الدورة الدموية
مواضيع عامة في علم الامراض
الحشرات
التقانة الإحيائية
مواضيع عامة في التقانة الإحيائية
التقنية الحيوية المكروبية
التقنية الحيوية والميكروبات
الفعاليات الحيوية
وراثة الاحياء المجهرية
تصنيف الاحياء المجهرية
الاحياء المجهرية في الطبيعة
أيض الاجهاد
التقنية الحيوية والبيئة
التقنية الحيوية والطب
التقنية الحيوية والزراعة
التقنية الحيوية والصناعة
التقنية الحيوية والطاقة
البحار والطحالب الصغيرة
عزل البروتين
هندسة الجينات
التقنية الحياتية النانوية
مفاهيم التقنية الحيوية النانوية
التراكيب النانوية والمجاهر المستخدمة في رؤيتها
تصنيع وتخليق المواد النانوية
تطبيقات التقنية النانوية والحيوية النانوية
الرقائق والمتحسسات الحيوية
المصفوفات المجهرية وحاسوب الدنا
اللقاحات
البيئة والتلوث
علم الأجنة
اعضاء التكاثر وتشكل الاعراس
الاخصاب
التشطر
العصيبة وتشكل الجسيدات
تشكل اللواحق الجنينية
تكون المعيدة وظهور الطبقات الجنينية
مقدمة لعلم الاجنة
الأحياء الجزيئي
مواضيع عامة في الاحياء الجزيئي
علم وظائف الأعضاء
الغدد
مواضيع عامة في الغدد
الغدد الصم و هرموناتها
الجسم تحت السريري
الغدة النخامية
الغدة الكظرية
الغدة التناسلية
الغدة الدرقية والجار الدرقية
الغدة البنكرياسية
الغدة الصنوبرية
مواضيع عامة في علم وظائف الاعضاء
الخلية الحيوانية
الجهاز العصبي
أعضاء الحس
الجهاز العضلي
السوائل الجسمية
الجهاز الدوري والليمف
الجهاز التنفسي
الجهاز الهضمي
الجهاز البولي
المضادات الحيوية
مواضيع عامة في المضادات الحيوية
مضادات البكتيريا
مضادات الفطريات
مضادات الطفيليات
مضادات الفايروسات
علم الخلية
الوراثة
الأحياء العامة
المناعة
التحليلات المرضية
الكيمياء الحيوية
مواضيع متنوعة أخرى
الانزيمات
Azurin
المؤلف:
E. T. Adman
المصدر:
Adv. Protein Chem. 42, 145–198
الجزء والصفحة:
9-12-2015
3856
Azurin
Azurins are proteins of bacterial origin that belong to the family of cupredoxins (ie, blue single-copper proteins) as is plastocyanin (1). Azurins consist of a single polypeptide chain that contains ca. 128 amino acid residues (~14 kDa molecular weight) (2). Several X-ray crystallography studies of azurins isolated from various bacteria, as well as of single-site mutated forms, have yielded high-resolution, three-dimensional protein structures (Fig. 1) (1, 3-6). The protein consists of eight b-strands that form a b-sandwich (barrel) structure. A segment between b-strands 4 and 5 lies outside this barrel and contains a short a-helix (residues 55 to 67). The copper ion is bound about 7 Å below the protein's surface and is coordinated by five ligands: three equatorial ones [a cysteine(112) thiolate and two imidazoles (His46 and His117)] and two weak ligands in axial positions (the carbonyl O atoms of Gly45 and Met121). This configuration (the carbonyl O atom in Gly45 excepted), is conserved in all known structures of “blue” type 1 (T1) copper sites determined thus far (1) .
Figure 1. The three-dimensional structure of azurin. The copper center with its ligands is seen near the top of the molecule. The disulfide bond is found at the opposite end of the molecule.
These protein–metal interactions yield a copper site that has a distorted, trigonal bipyramidal geometry, which may explain the stabilization of the Cu(I) state relative to Cu(II) (7) (see Redox Enzymes). Structure-imposed Cu → L p-backbonding has been proposed to account further for stabilization of the cuprous state, because strong p-interaction with the dp orbitals results in an increase of the ligand field strength. The oxidation/reduction potentials of the Cu(II)/Cu(I) in azurins( ~310 mV) are higher than is generally observed for copper complexes. Studies of several mutant forms in which Met121 is replaced, demonstrated that large hydrophobic residues raise the Cu(II)/ (I) potential, whereas negatively charged ones lower it (8): The span in reduction potential going from Glu121 to Leu121 azurin is 300 mV, or about half the range of the potentials for the naturally occurring T1 sites. The large increase in reduction potentials caused by substitutions involving bulky hydrophobic side chains at position 121 is rationalized by exclusion by such side chains of water from the metal site or simply by providing a low dielectric environment for the copper ion. In contrast, negatively charged hydrophilic residues or water are expected to stabilize the copper ion in the more positively charged +2 state and thus lower the potential, as is indeed observed. Smaller effects on the reduction potential were observed when mutations were made in other positions. It should be emphasized, however, that a thiolate copper ligand per se is not a prerequisite for high reduction potentials (8).
As with all other cupredoxins, azurins are also spectroscopically characterized by an intense blue color and a uniquely narrow hyperfine coupling in the electron paramagnetic resonance (EPR(spectrum. The intense blue color is due to a ligand-to-metal charge transfer involving the thiolate ligand as the electron donor; it has a molar extinction coefficient of 4000 to 6000 M–1cm–1 (9). This is more than 100 times larger than what is found for simple Cu(II) complexes. A second characteristic property associated with the blue (T1) Cu (II) is an EPR spectrum displaying a hyperfine splitting in the g region, due to interaction of the Cu(II) nuclear and electron spins, which is unusually narrow (~0.008 cm–1), or approximately 50% smaller than those of inorganic copper complexes. This is attributed to delocalization of the unpaired electron onto the Cys(S) pp orbital, thus reducing the nuclear–electron interaction (10). The unique spectroscopic properties of the T1 site are most likely related to the electron-mediating function of cupredoxins, and this relationship is a central issue in studies of biological electron transfer proteins (11).
Recent experimental investigations of the electron transfer mechanism by azurin have in part been directed toward the intramolecular process in single-site mutated forms of the proteins (12-14). This was motivated by the finding that azurin provides a useful model system for studies of long-range, intraprotein electron transfer (LRET). One way by which this reaction can be induced is from the half-reduced disulfide bond to the Cu(II) ion (12, 14). Another one is by attaching an extraneous redox center to the protein (11, 13). Results of these studies yielded insights into the mechanisms of intramolecular electron transfer in proteins and the parameters that determine the degree of electronic coupling between electron donor and acceptor. Effective electron transfer in proteins requires minimal reorganization of the redox centers as a result of the process. As described above, the metal ion in the blue copper proteins is coordinated to a site that is intermediate between the preferred geometries for Cu(II) (tetragonal planar) and Cu(I) (tetrahedral) (6). Furthermore, the relatively high reduction potentials provides an increased driving force. Finally, the tightly knit, antiparallel b-sheet structure of azurin probably improves the electronic coupling between the reaction partners through the protein matrix.
References
1. E. T. Adman (1991) Adv. Protein Chem. 42, 145–198.
2. L. Ryden and J.-O. Lundgren (1976) Nature 261, 344–346.
3. E. T. Adman and L. H. Jensen (1981) Isr. J. Chem. 21, 8–12.
4. H. Nar, A. Messerschmidt, and R. Huber (1991) J. Mol. Biol. 218, 427–447.
5. W. E. B. Shephard, B. F. Anderson, D. A. Lewandoski, G. E. Norris, and E. N. Baker (1990) J. Am. Chem. Soc. 112, 7817–7819.
6. A. Messerschmidt, L. Prade, S. J. Kroes, J. Sanders-Loehr, R. Huber, and G. W. Canters (1998) Proc. Natl. Acad. Sci. USA 95, 3443–3448.
7. C. S. St. Clair, W. R. Ellis Jr., and H. B. Gray (1992) Inorg. Chim. Acta 191, 149–155.
8. T. Pascher, B. G. Karlsson, M. Nordling, B. G. Malmström, and T. Vanngård (1993) Eur. J. Biochem. 212, 289–296.
9. H. B. Gray and E. I. Solomon (1981) In Copper Proteins, Vol. 3 (T. G. Spiro, ed.), Wiley, New York, pp. 1–39.
10. W. E. Antholine, P. M. Hanna, and D. R. McMillin (1993) Biophys. J. 64, 267–272.
11. A. J. Di Bilio et al. (1997) J. Am. Chem. Soc. 119, 9921–9922.
12. O. Farver, L. K. Skov, G. Gilardi, G. van Pouderoyen, G. W. Canters, S. Wherland, and I. Pecht (1996) Chem. Phys. 204, 271–277.
13. J. J. Regan et al. (1995) Chem. Biol. 2, 489–496.
14. O. Farver and I. Pecht (1997) J. Biol. Inorg. Chem. 2, 387–392.
15. T. J. Mizoguchi, A. J. Di Bilio, H. B. Gray, and J. H. Richards (1992) J. Am. Chem. Soc. 114, 10076- 10078 .
الاكثر قراءة في مواضيع عامة في الاحياء الجزيئي
اخر الاخبار
اخبار العتبة العباسية المقدسة
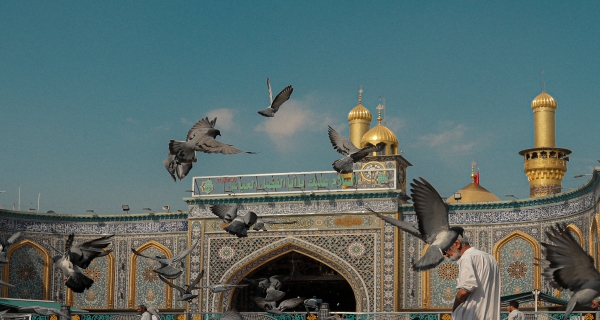
الآخبار الصحية
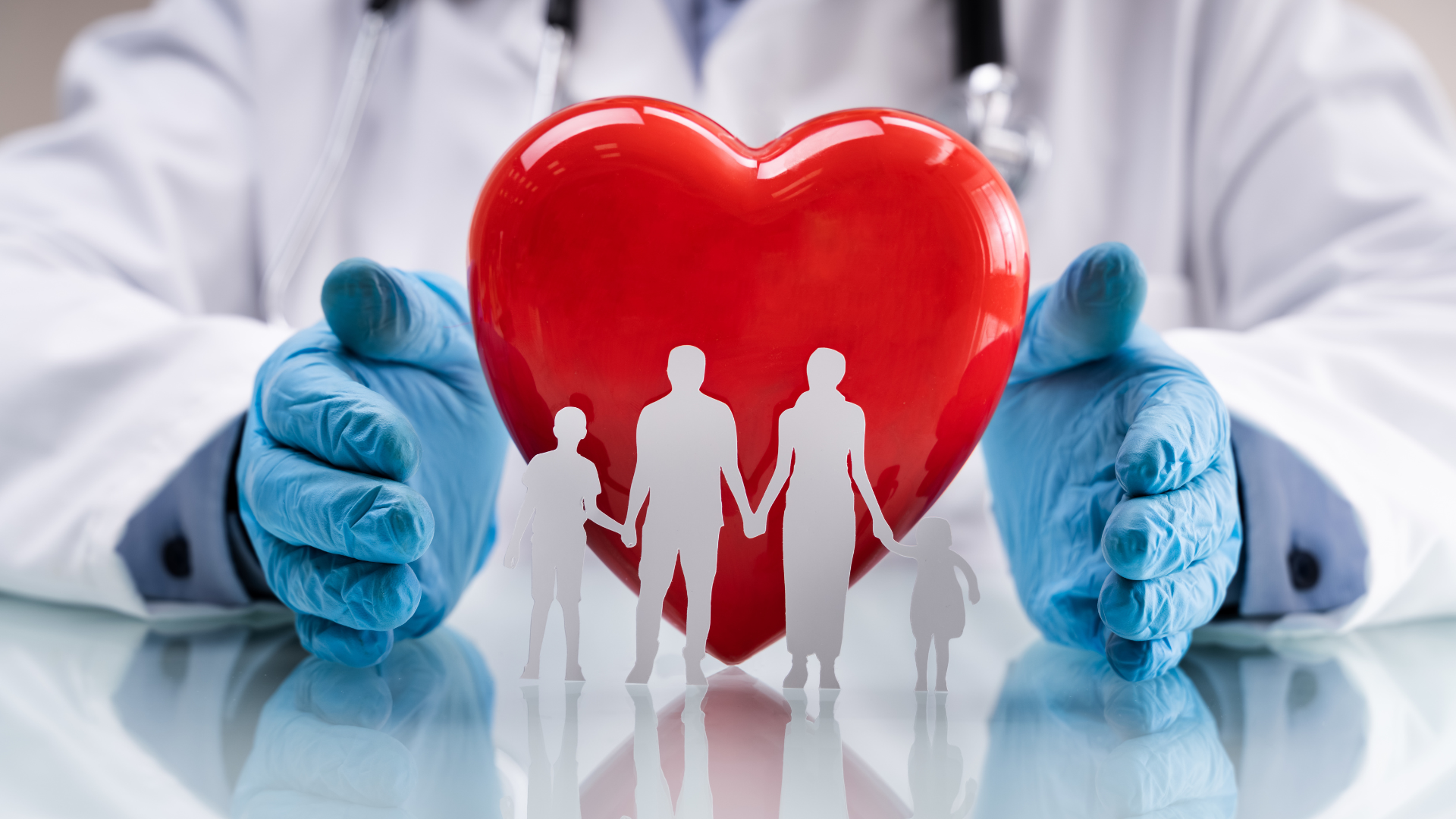