النبات
مواضيع عامة في علم النبات
الجذور - السيقان - الأوراق
النباتات الوعائية واللاوعائية
البذور (مغطاة البذور - عاريات البذور)
الطحالب
النباتات الطبية
الحيوان
مواضيع عامة في علم الحيوان
علم التشريح
التنوع الإحيائي
البايلوجيا الخلوية
الأحياء المجهرية
البكتيريا
الفطريات
الطفيليات
الفايروسات
علم الأمراض
الاورام
الامراض الوراثية
الامراض المناعية
الامراض المدارية
اضطرابات الدورة الدموية
مواضيع عامة في علم الامراض
الحشرات
التقانة الإحيائية
مواضيع عامة في التقانة الإحيائية
التقنية الحيوية المكروبية
التقنية الحيوية والميكروبات
الفعاليات الحيوية
وراثة الاحياء المجهرية
تصنيف الاحياء المجهرية
الاحياء المجهرية في الطبيعة
أيض الاجهاد
التقنية الحيوية والبيئة
التقنية الحيوية والطب
التقنية الحيوية والزراعة
التقنية الحيوية والصناعة
التقنية الحيوية والطاقة
البحار والطحالب الصغيرة
عزل البروتين
هندسة الجينات
التقنية الحياتية النانوية
مفاهيم التقنية الحيوية النانوية
التراكيب النانوية والمجاهر المستخدمة في رؤيتها
تصنيع وتخليق المواد النانوية
تطبيقات التقنية النانوية والحيوية النانوية
الرقائق والمتحسسات الحيوية
المصفوفات المجهرية وحاسوب الدنا
اللقاحات
البيئة والتلوث
علم الأجنة
اعضاء التكاثر وتشكل الاعراس
الاخصاب
التشطر
العصيبة وتشكل الجسيدات
تشكل اللواحق الجنينية
تكون المعيدة وظهور الطبقات الجنينية
مقدمة لعلم الاجنة
الأحياء الجزيئي
مواضيع عامة في الاحياء الجزيئي
علم وظائف الأعضاء
الغدد
مواضيع عامة في الغدد
الغدد الصم و هرموناتها
الجسم تحت السريري
الغدة النخامية
الغدة الكظرية
الغدة التناسلية
الغدة الدرقية والجار الدرقية
الغدة البنكرياسية
الغدة الصنوبرية
مواضيع عامة في علم وظائف الاعضاء
الخلية الحيوانية
الجهاز العصبي
أعضاء الحس
الجهاز العضلي
السوائل الجسمية
الجهاز الدوري والليمف
الجهاز التنفسي
الجهاز الهضمي
الجهاز البولي
المضادات الحيوية
مواضيع عامة في المضادات الحيوية
مضادات البكتيريا
مضادات الفطريات
مضادات الطفيليات
مضادات الفايروسات
علم الخلية
الوراثة
الأحياء العامة
المناعة
التحليلات المرضية
الكيمياء الحيوية
مواضيع متنوعة أخرى
الانزيمات
AP Endonucleases
المؤلف:
M. L. Dodson, R. D. Schrock, and R. S. Lloyd
المصدر:
Biochemistry 32, 8284–8290.
الجزء والصفحة:
7-12-2015
4148
AP Endonucleases
AP endonucleases are enzymes that cleave the phosphodiester bond on either side of an AP site in DNA and are involved in base excision repair. Enzymes that cleave the phosphodiester bond to the 3′ side are referred to as type I AP endonucleases, and those that cleave the phosphodiester bond 5′ to the AP site are type II AP endonucleases. Whether or not a bona fide type I AP endonuclease exists that is not associated with DNA glycosylase activity is not known. However, type II AP endonucleases, which cleave AP sites by hydrolysis, have been found in many organisms and have been characterized.
1. AP Lyases (Class I AP Endonucleases(
Type I AP endonucleases are also DNA glycosylases, and it appears that the same active site is involved in both the glycosylase and AP endonuclease activities. However, it has also been demonstrated that cleavage of AP site occurs by b-elimination, which involves abstraction of a hydrogen from the C2′ position of the deoxyribose. Because this is a lyase reaction, and not a hydrolyase reaction, the type I AP endonucleases are referred to as AP lyases (1).
Endonuclease III and 8-oxoguanine glycosylase are examples of this class of enzymes (1, 2). The a-NH2 group of the N-terminal amino acid residue forms a Schiff base with the ring-opened form of the deoxyribose, eventually leading to cleavage of the deoxyribose–phosphate bond without water addition. Following elimination of the base by glycosylase action, the enzyme remains attached to DNA through a protonated Schiff base intermediate where the C1′ of the sugar is covalently bound to the nitrogen of the N-terminal a-amino group of the protein (3). b-Elimination of this intermediate, followed by hydrolysis of the Schiff base, results in cleavage of the phosphodiester bond and generation of a trans a–b-unsaturated aldehyde on the 5′ side, and a 5′-phosphate on the 3′ side, of the cleavage, which is concomitant with the release of the enzyme. When the AP lyase activity is associated with a glycosylase activity, the two reactions are, for the most part, coupled (2). In these instances, it is natural to assign a physiological role to the AP lyase. However, cleavage of an AP site on the 3′ side occurs readily even in the absence of enzymes and can be accelerated by alkaline pH and by basic proteins such as histones and cytochrome c. Hence the assignment of AP lyase activity to a protein in the absence of an associated glycosylase activity is virtually impossible.
2. AP Endonucleases (Class II AP Endonucleases(
These enzymes hydrolyze the phosphodiester bond 5′ to an AP site. AP endonuclease IV in E. coli, which has a homologue in yeast and humans, is the only example of a “pure” AP endonuclease—that is, an endonuclease with only hydrolysis activity 5′ to an AP site and with no significant activity on any other substrate. However, the best-characterized AP endonuclease is the E. coli exonuclease III and its homologues in eukaryotes, including yeast, Drosophila, and mammals. In contrast to the pure AP nuclease endonuclease IV, the exonuclease III, as the name implies, has exonuclease activity as well.
2.1. Exonuclease III/Rrp1/APE
The prototype of this group of enzymes, exonuclease III, has several activities, including ribonuclease H, 3′ to 5′ exonuclease, and type II AP endonuclease. The enzyme is specific for double-stranded DNA. It is a simple polypeptide chain of 30 kDa with no cofactor. The X-ray crystallography structure of an enzyme–substrate complex demonstrates that the base is flipped out of the double helix, into the active site of the enzyme (4). The E. coli enzyme is a potent 3′ to 5′ exonuclease. The exonuclease activity has a preference for blunt double-stranded termini, but it can initiate exonuclease action from a nick as well. An activity related to the 3′ to 5′ exonuclease function is the ability of the enzyme to remove deoxyribose fragments, including 3′-phosphoglycolate esters and 3′-phosphate, from the 3′ termini of DNA strand breaks generated by attack of reactive oxygen species on DNA. Exonuclease III-defective mutants are extremely sensitive to H2O2 and ionizing radiation, which most probably kills cells through the strand breaks introduced by reactive oxygen species. As a rule, these are not clean breaks with 3′-OH and 5′-P termini. Instead, they most often contain either 3′-phosphate or fragmented deoxyribose, which must be processed further before they can be utilized by DNA polymerases to fill in the gap created by the DNA damage. These structures are removed efficiently by exonuclease III, but not by endonuclease IV.
The Drosophila exonuclease III homologue is a monomer of 75 kDa. The 250-amino-acid-residue C-terminal region of the protein is homologous to the exonuclease III family of AP endonucleases. However, the N-terminal 400 residues are not related to any known sequence, and the function of this putative domain is unknown at present. The Drosophila enzyme was initially isolated as an activity that catalyzes strand transfer and was thought to perform a RecA-like function in this organism, hence the name Rrp1 (Recombination related protein 1). However, later work has not confirmed a role for this protein in recombination. The enzyme has a potent type II AP endonuclease activity, but its 3′ to 5′ exonuclease activity is rather modest compared to the E. coli enzyme and is only 10–2 to 10–3 of the AP endonuclease activity. Whether the N-terminal 400 residues play any role in recombination or in DNA repair is not known at present.
The human AP endonuclease (APE/HAP1/APEX) is highly homologous (90% to 95% sequence identity) to the enzymes from bovine and rodent sources. These enzymes are 35-kDa monomers and are clearly related to E. coli exonuclease III. The mammalian enzymes have potent type II AP endonuclease and 3′-phosphoglycol aldehyde esterase activities necessary for denuding a “jagged” 3′ terminus caused by direct attack of reactive oxygen species or by direct hit by ionizing radiation. In contrast to exonuclease III, however, the 3′ to 5′ exonuclease activity of mammalian type II AP endonucleases is rather weak and undetectable with certain 3′ sequences. The structure of human AP endonuclease indicates that it flips out the deoxyribose moiety into the active site (5), as is observed also in the E. coli exonuclease III.
A unique property of mammalian APE proteins is their capacity to reduce critical cysteine residues in certain transcription factors and by doing so activate these transcription factors. In fact, the human APE was also purified as a factor that activates the AP-1(Jun/Fos) transcription factor and was named Ref (for reducing factor 1) before the realization that it is identical to APE. The APE cysteine residue involved in this reduction reaction is located in the NH2-terminal region and plays no role in the AP endonuclease activity of this protein. In addition to Jun and Fos, other transcription factors, including USF, NF-kB, c-Myb, and v-Rel, are activated by reduction of an oxidized cysteine by APE in vitro (5). However, the physiological role of these in vitro activities is currently not known.
Mouse APE knockout mutants are lethal at the embryonic stage. This could be due to either (a) the importance of base excision repair for survival of the organism or (b) disruption of an activating mechanism for important transcription factors.
2.2. Endonuclease IV
The E. coli endonuclease IV, Nfo, is a monomer of 30 kDa that cleaves 5′ to AP sites in a reaction that is independent of divalent metal ions such as Mg2+ . It also has potent 3′-PGA diesterase and 3′-phosphatase activities but does not have exonuclease function. Although Nfo– mutants are not especially sensitive to H2O2, they are hypersensitive to killing by the oxidative agents bleomycin and t-butylhydroperoxide, indicating that endonuclease IV acts on a subclass of oxidative DNA lesions that are not processed efficiently by exonuclease III. The importance of Nfo in cellular defense against oxidative stress is underscored by the fact that this is the only DNA repair enzyme that is induced by oxidative stress as part of the SoxRS regulon.
The Nfo homologue in yeast, Apn1, is the major AP endonuclease in this organism. Apn1 is a monomer of 40 kDa with 31% sequence identity with the E. coli Nfo endonuclease. The biochemical properties of Apn1 are very similar to those of Nfo, and indeed the expression of Apn1 in E. coli corrects the oxidant-sensitive phenotype of nfo mutants, but not the H2O2 sensitivity.
References
1. V. Bailly and W. G. Verly (1987) Biochem. J. 242, 565–572.
2. M. L. Dodson, R. D. Schrock, and R. S. Lloyd (1993) Biochemistry 32, 8284–8290.
3. R. P. Cunningham, H. Asahara, J. F. Bank, C. P. Scholes, J. C. Salerno, K. Surerus, E. Munck, J. McCracken, J. Peisach, and M. H. Emptage (1988) Biochemistry 28, 4450–4455.
4. C. D. Mol, C. F. Kuo, M. M. Thayer, R. P. Cunningham, and J. A. Tainer (1995) Nature 374 381–386 ,
5. M. A. Gorman, S. Morera, D. G. Rothwell, E. de la Fortelle, C. D. Mol, J. A. Tainer, I. D. Hickson, and P. S. Freemont (1997) EMBO J. 16, 6548–6558.
الاكثر قراءة في مواضيع عامة في الاحياء الجزيئي
اخر الاخبار
اخبار العتبة العباسية المقدسة
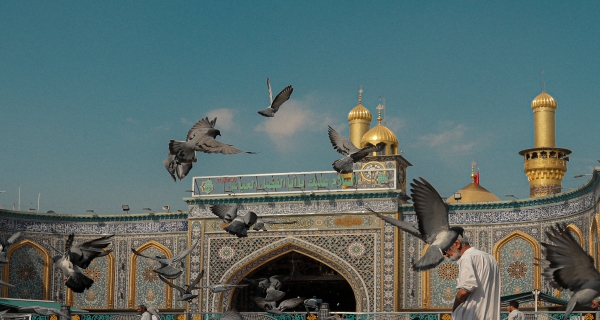
الآخبار الصحية
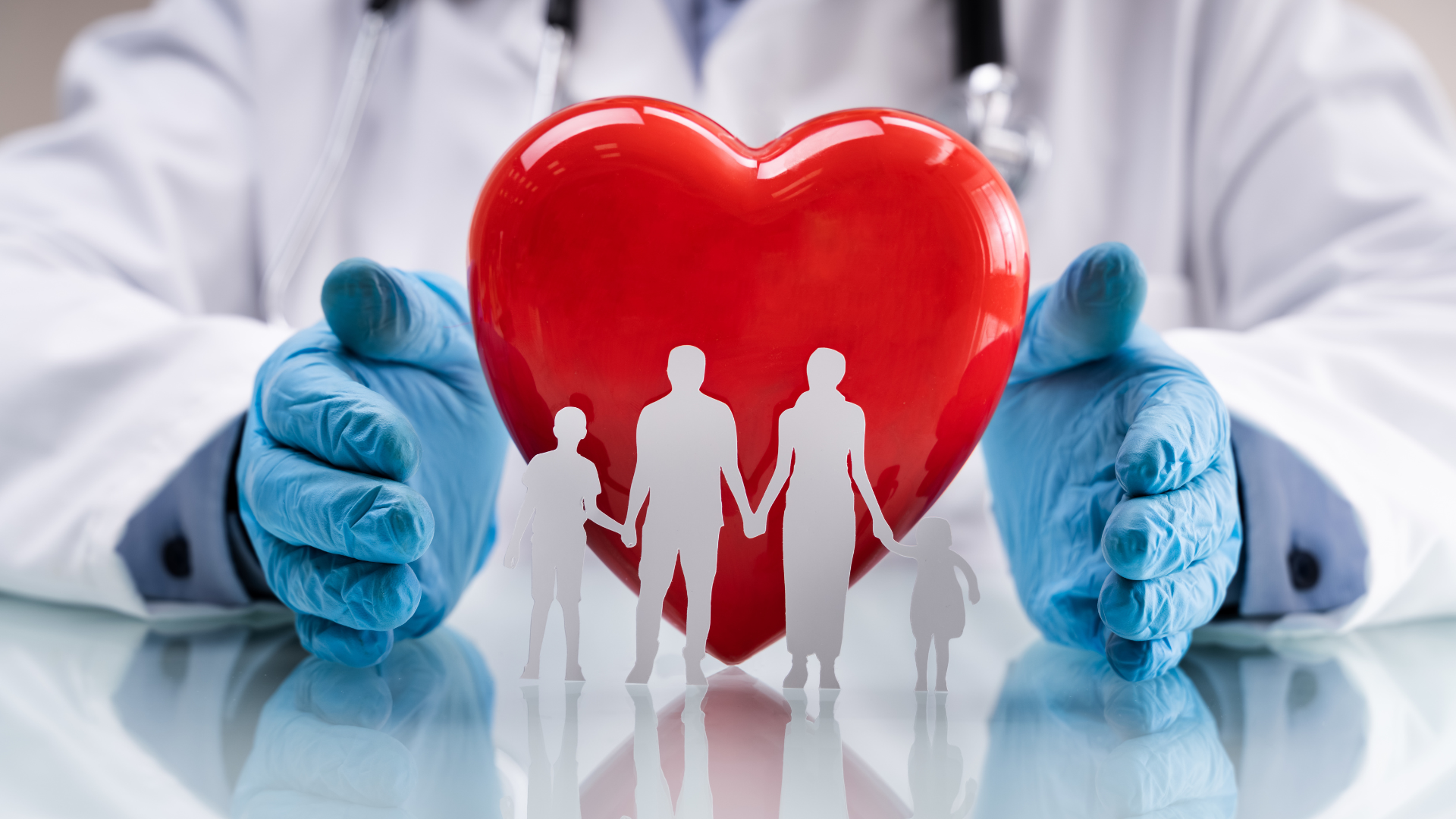