النبات
مواضيع عامة في علم النبات
الجذور - السيقان - الأوراق
النباتات الوعائية واللاوعائية
البذور (مغطاة البذور - عاريات البذور)
الطحالب
النباتات الطبية
الحيوان
مواضيع عامة في علم الحيوان
علم التشريح
التنوع الإحيائي
البايلوجيا الخلوية
الأحياء المجهرية
البكتيريا
الفطريات
الطفيليات
الفايروسات
علم الأمراض
الاورام
الامراض الوراثية
الامراض المناعية
الامراض المدارية
اضطرابات الدورة الدموية
مواضيع عامة في علم الامراض
الحشرات
التقانة الإحيائية
مواضيع عامة في التقانة الإحيائية
التقنية الحيوية المكروبية
التقنية الحيوية والميكروبات
الفعاليات الحيوية
وراثة الاحياء المجهرية
تصنيف الاحياء المجهرية
الاحياء المجهرية في الطبيعة
أيض الاجهاد
التقنية الحيوية والبيئة
التقنية الحيوية والطب
التقنية الحيوية والزراعة
التقنية الحيوية والصناعة
التقنية الحيوية والطاقة
البحار والطحالب الصغيرة
عزل البروتين
هندسة الجينات
التقنية الحياتية النانوية
مفاهيم التقنية الحيوية النانوية
التراكيب النانوية والمجاهر المستخدمة في رؤيتها
تصنيع وتخليق المواد النانوية
تطبيقات التقنية النانوية والحيوية النانوية
الرقائق والمتحسسات الحيوية
المصفوفات المجهرية وحاسوب الدنا
اللقاحات
البيئة والتلوث
علم الأجنة
اعضاء التكاثر وتشكل الاعراس
الاخصاب
التشطر
العصيبة وتشكل الجسيدات
تشكل اللواحق الجنينية
تكون المعيدة وظهور الطبقات الجنينية
مقدمة لعلم الاجنة
الأحياء الجزيئي
مواضيع عامة في الاحياء الجزيئي
علم وظائف الأعضاء
الغدد
مواضيع عامة في الغدد
الغدد الصم و هرموناتها
الجسم تحت السريري
الغدة النخامية
الغدة الكظرية
الغدة التناسلية
الغدة الدرقية والجار الدرقية
الغدة البنكرياسية
الغدة الصنوبرية
مواضيع عامة في علم وظائف الاعضاء
الخلية الحيوانية
الجهاز العصبي
أعضاء الحس
الجهاز العضلي
السوائل الجسمية
الجهاز الدوري والليمف
الجهاز التنفسي
الجهاز الهضمي
الجهاز البولي
المضادات الحيوية
مواضيع عامة في المضادات الحيوية
مضادات البكتيريا
مضادات الفطريات
مضادات الطفيليات
مضادات الفايروسات
علم الخلية
الوراثة
الأحياء العامة
المناعة
التحليلات المرضية
الكيمياء الحيوية
مواضيع متنوعة أخرى
الانزيمات
Recombination Occurs by Physical Exchange of DNA
المؤلف:
JOCELYN E. KREBS, ELLIOTT S. GOLDSTEIN and STEPHEN T. KILPATRICK
المصدر:
LEWIN’S GENES XII
الجزء والصفحة:
1-3-2021
2591
Recombination Occurs by Physical Exchange of DNA
KEY CONCEPTS
-Recombination is the result of crossing over that occurs at a chiasma during meiosis and involves two of the four chromatids of the tetrad.
-Recombination occurs by a breakage and reunion that proceeds via an intermediate of heteroduplex DNA that depends on the complementarity of the two strands of DNA.
-The frequency of recombination between two genes is proportional to their physical distance; Recombination between genes that are very closely linked is rare.
-For genes that are very far apart on a single chromosome, the frequency of recombination is not proportional to their physical distance because recombination happens so frequently.
The term genetic recombination describes the generation of new combinations of alleles at each generation in diploid organisms. This arises because the two homologous copies of each chromosome might have different alleles at some loci. By the exchange of corresponding segments between the homologs, called crossing over, recombinant chromosomes that are different from the parental chromosomes can be generated.
Recombination results from a physical exchange of chromosomal material. For example, recombination might result from the crossing over that occurs when homologous chromosomes align during meiosis (the specialized division that produces haploid gametes).
Meiosis begins with a cell that has duplicated its chromosomes so that it has four copies of each chromatid (the two homologous chromosomes and their identical [sister] copies that remain joined after duplication). Early in meiosis, all four chromatids are closely associated (synapsed) in a structure called a bivalent and, later, a tetrad. At this point, pairwise exchanges of material between two nonidentical (nonsister) chromatids (of the four total) can occur.
The point of synapsis between homologs is called a chiasma; this is illustrated diagrammatically in FIGURE 1. A chiasma represents a site at which one DNA strand in each of two nonsister chromatids in a tetrad has been broken and exchanged. If during the resolution of the chiasma the previously unbroken strands are also broken and exchanged, recombinant chromatids will be generated. Each recombinant chromatid consists of material derived from one chromatid on one side of the chiasma, with material from the other chromatid on the opposite side. The two recombinant chromatids have reciprocal structures. The event is described as a “breakage and reunion.” Because each individual crossing-over event involves only two of the four associated chromatids, a single recombination event can produce only 50% recombinants.
FIGURE 1. Chiasma formation at Prophase I of meiosis is responsible for generating recombinant chromosomes.
The complementarity of the two strands of DNA is essential for the recombination process. Each of the chromatids shown in Figure consists of a very long duplex of DNA. For them to be broken and reconnected without any loss of material requires a mechanism to recognize and align at exactly corresponding positions; this mechanism is complementary base pairing.
Recombination results from a process in which the single strands in the region of the crossover exchange their partners, resulting in a branch that might migrate for some distance in either direction.
FIGURE 2. shows that this creates a stretch of heteroduplex DNA in which the single strand of one duplex is paired with its complement from the other duplex. Each duplex DNA corresponds to one of the chromatids involved in recombination in Figure 1.
The mechanism, of course, involves other stages in which strands must be broken and religated, which we discuss in more detail in the chapter titled Homologous and Site-Specific Recombination, but the crucial feature that makes precise recombination possible is the complementarity of DNA strands. Figure 2. shows only some stages of the reaction, but we see that a stretch of heteroduplex DNA forms in the recombination intermediate when a single strand crosses over from one duplex to the other. Each recombinant consists of one parental duplex DNA at the left, which is connected by a stretch of heteroduplex DNA to the other parental duplex at the right.
FIGURE 2. Recombination involves pairing between complementary strands of the two parental duplex DNAs.
The formation of heteroduplex DNA requires the sequences of the two recombining duplexes to be close enough to allow pairing between the complementary strands. If there are no differences between the two parental genomes in this region, formation of heteroduplex DNA will be perfect. However, pairing can still occur even when there are small differences. In this case, the heteroduplex DNA has points of mismatch, at which a base in one strand is paired with a base in the other strand that is not complementary to it. The correction of such mismatches is another feature of genetic recombination .
Over chromosomal distances, recombination events occur more or less at random with a characteristic frequency. The probability that a crossover will occur within any specific region of the chromosome is more or less proportional to the length of the region, up to a saturation point. For example, a large human chromosome usually has three or four crossover events per meiosis, whereas a small chromosome might have only one on average.
FIGURE 1.39 compares recombination frequencies in three situations: two genes on different chromosomes, two genes that are far apart on the same chromosome, and two genes that are close together on the same chromosome. Genes on different chromosomes segregate independently according to Mendel’s principles, resulting in the production of 50% “parental” types and 50% “recombinant” types during meiosis. When genes are sufficiently far apart on the same chromosome, the probability of at least one crossover in the region between them becomes so high that their association is the same as that of genes on different chromosomes and they show 50% recombination.
FIGURE 3. Genes on different chromosomes segregate independently so that all possible combinations of alleles are produced in equal proportions. Crossing over occurs so frequently between genes that are far apart on the same chromosome that they effectively segregate independently. But recombination is reduced when genes are closer together, and for adjacent genes it might hardly ever occur.
When genes are close together, though, the probability of a crossover between them is reduced, and recombination occurs only in some proportion of meioses. For example, if it occurs in onequarter of the meioses, the overall rate of recombination is 12.5% (because a single recombination event produces 50% recombination, and this occurs in 25% of meioses). When genes are very close together, as shown in the bottom panel of Figure 3, recombination between them might never be observed in phenotypes of multicellular eukaryotes (because they produce few offspring).
This leads us to the concept that a chromosome is an array of many genes. Each protein-coding gene is an independent unit of expression and is represented in one or more polypeptide chains.
The properties of a gene can be changed by mutation. The allelic combinations present on a chromosome can be changed by recombination. We can now ask, “What is the relationship between the sequence of a gene and the sequence of the polypeptide chain it encodes?”
الاكثر قراءة في مواضيع عامة في الاحياء الجزيئي
اخر الاخبار
اخبار العتبة العباسية المقدسة
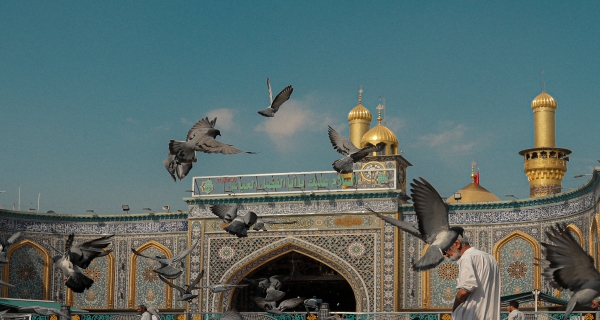
الآخبار الصحية
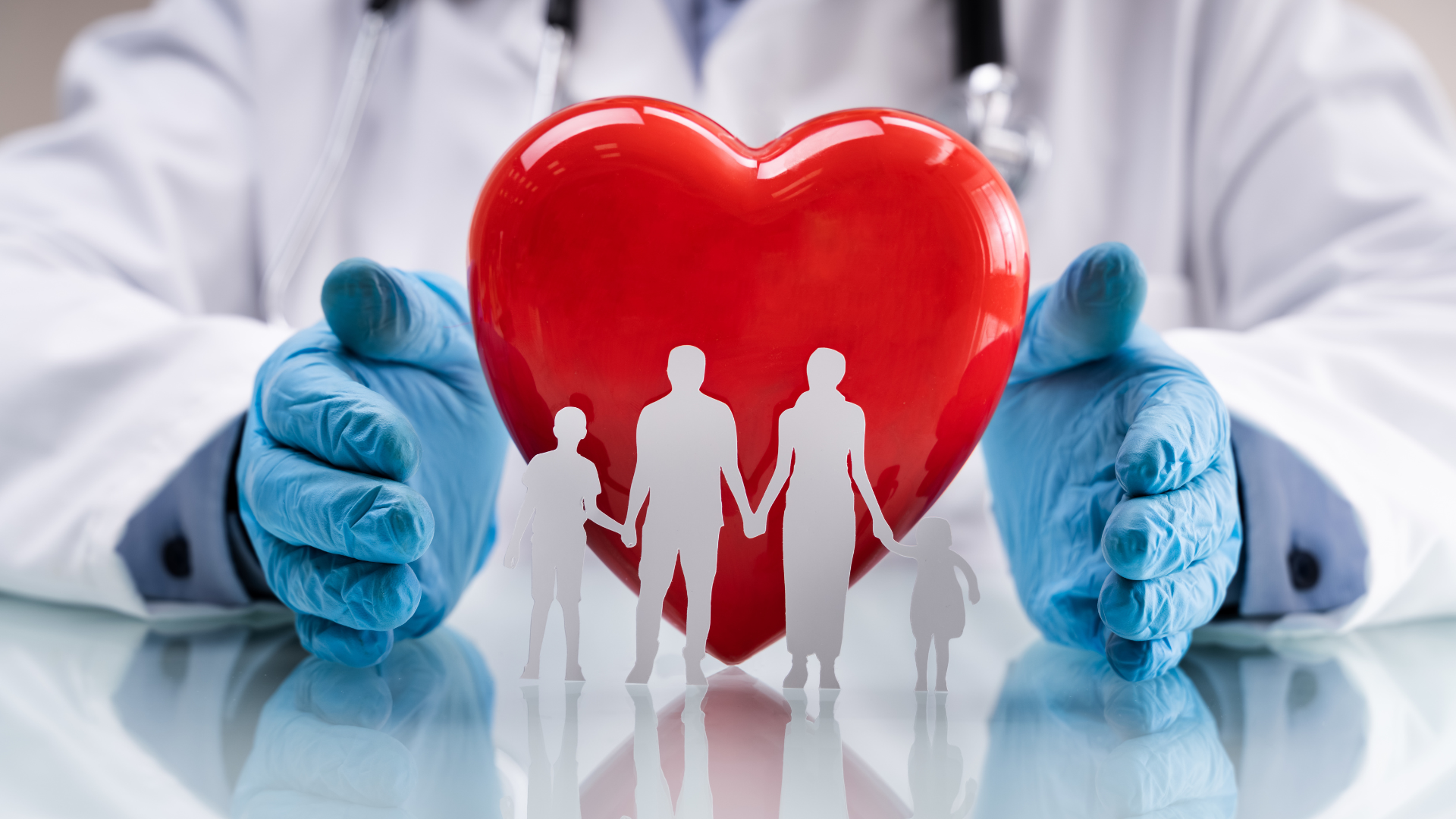