تاريخ الفيزياء
علماء الفيزياء
الفيزياء الكلاسيكية
الميكانيك
الديناميكا الحرارية
الكهربائية والمغناطيسية
الكهربائية
المغناطيسية
الكهرومغناطيسية
علم البصريات
تاريخ علم البصريات
الضوء
مواضيع عامة في علم البصريات
الصوت
الفيزياء الحديثة
النظرية النسبية
النظرية النسبية الخاصة
النظرية النسبية العامة
مواضيع عامة في النظرية النسبية
ميكانيكا الكم
الفيزياء الذرية
الفيزياء الجزيئية
الفيزياء النووية
مواضيع عامة في الفيزياء النووية
النشاط الاشعاعي
فيزياء الحالة الصلبة
الموصلات
أشباه الموصلات
العوازل
مواضيع عامة في الفيزياء الصلبة
فيزياء الجوامد
الليزر
أنواع الليزر
بعض تطبيقات الليزر
مواضيع عامة في الليزر
علم الفلك
تاريخ وعلماء علم الفلك
الثقوب السوداء
المجموعة الشمسية
الشمس
كوكب عطارد
كوكب الزهرة
كوكب الأرض
كوكب المريخ
كوكب المشتري
كوكب زحل
كوكب أورانوس
كوكب نبتون
كوكب بلوتو
القمر
كواكب ومواضيع اخرى
مواضيع عامة في علم الفلك
النجوم
البلازما
الألكترونيات
خواص المادة
الطاقة البديلة
الطاقة الشمسية
مواضيع عامة في الطاقة البديلة
المد والجزر
فيزياء الجسيمات
الفيزياء والعلوم الأخرى
الفيزياء الكيميائية
الفيزياء الرياضية
الفيزياء الحيوية
الفيزياء العامة
مواضيع عامة في الفيزياء
تجارب فيزيائية
مصطلحات وتعاريف فيزيائية
وحدات القياس الفيزيائية
طرائف الفيزياء
مواضيع اخرى
Robert Mayer
المؤلف:
William H. Cropper
المصدر:
Great Physicists
الجزء والصفحة:
9-12-2015
2954
Robert Mayer
Something is Conserved
To the modern student, the term energy has a meaning that is almost self-evident. This meaning was far from clear, however, to scientists of the early nineteenth century. The many effects that would finally be unified by the concept of energy were still seen mostly as diverse phenomena. It was suspected that mechanical, thermal, chemical, electrical, and magnetic effects had something in common, but the connections were incomplete and confused.
What was most obvious by the 1820s and 1830s was that strikingly diverse effects were interconvertible. Alessandro Volta’s electric cell, invented in 1800, produced electrical effects from chemical effects. In 1820, Hans Christian Oersted observed magnetic effects produced by electrical effects. Magnetism produces motion (mechanical effects), and for many years it had been known that motion can produce electrical effects through friction. This sequence is a chain of “conversions”:
Chemical effect → electrical effect → magnetic effect → mechanical effect → electrical effect.
In 1822, Thomas See beck demonstrated that a bimetallic junction produces an electrical effect when heated, and twelve years later Jean Peltier reported the reverse conversion: cooling produced by an electrical effect. Heat engines perform as conversion devices, converting a thermal effect (heat) into a mechanical effect (work).
Most of the major theories of science have been discovered by one scientist, or at most by a few. The search for broad theoretical unities tends to be difficult, solitary work, and important scientific discoveries are usually subtle enough that special kinds of genius are needed to recognize and develop them. But, as Thomas Kuhn points out, there is at least one prominent exception to this rule. The theoretical studies inspired by the discoveries of conversion processes, which finally gave us the energy concept, were far from a singular effort. Kuhn lists twelve scientists who contributed importantly during the early stages of this “simultaneous discovery.”
The idea that occurred to all twelve not quite simultaneously, but independently was that conversion was somehow linked with conservation. When one effect was converted to another, some measure of the first effect was quantitatively replaced by the same kind of measure of the second. This measure, applicable to all the various interconvertible effects, was conserved: throughout a conversion process its total amount, whether it assessed one effect, the other effect, or both, was precisely constant.
The twelve simultaneous discoverers were not the first to make important use of a conservation principle. In one form or another, conservation principles had been popular, almost intuitive it seems, with scientists for many years. Theorists had counted among their most impressive achievements discoveries of quantities that were both indestructible and un creatable. Adherents of the caloric theory of heat had postulated conservation of heat. In the late eighteenth century, Antoine- Laurent Lavoisier and others had established that mass is conserved in chemical reactions; when a chemical reaction proceeds in a closed container, there is no change in total mass.
So it was natural for theorists who studied conversion processes to attempt to build their theories from a conservation law. But, as always in the formulation of a conservation principle, a difficult question had to be asked at the outset: what is the quantity conserved? As it turned out, a workable answer to this question was practically impossible without some knowledge of the conservation law itself, because the most obvious property of the conserved quantity, ultimately identified as energy, was that it was conserved. No direct measurement like that of mass could be made for verification of the conservation property. This was a search for something that could not be fully defined until it was actually found.
Voyage of Discovery
One of the first to penetrate this conceptual tangle was Robert Mayer, a German physician and physicist who spent most of his life in Heilbronn, Germany. Mayer was a contemporary of James Joule, and like Joule, he was an amateur in the scientific fields that most absorbed his interest. His university training was in medicine, and what is known of his student record at the University of Tubingen shows little sign of intellectual genius. He was good at billiards and cards, devoted to his fraternity, and inclined to be rebellious and unpopular with the university authorities; eventually he was suspended for a year. With hindsight, we can see in Mayer’s reaction to the suspension a six-day hunger strike evidence for his stubbornness and sensitivity to criticism, and even some forewarning of his later mental problems.
Mayer’s youthful behavior was not that of an unmitigated rebel, however; when the Tubingen authorities permitted, he returned, finished his dissertation, and passed the doctoral examination. But he was still too restless to plan his future according to conventional (and family) expectations. Instead of settling into a routine medical practice, he decided to travel by taking a position as ship’s surgeon on a Dutch vessel sailing for the East Indies. He found little inspiration on this trip, either in the company of his fellow officers or in the quality and quantity of the ship’s food. But to Mayer the voyage was worth any amount of hunger and boredom.
Mayer tells us, in an exotic tale of scientific imagination, of an event in Java that set him on the intellectual path he followed for the rest of his life. On several occasions in 1840, when he let blood from sailors in an East Java port, Mayer noticed that venous blood had a surprisingly bright red color. He surmised that this unusual redness of blood in the tropics indicated a slower rate of metabolic oxidation. He became convinced that oxidation of food materials produced heat internally and maintained a constant body temperature. In a warm climate, he reasoned, the oxidation rate was reduced.
For those of us who are inclined toward the romantic view that theoreticians make their most inspired advances in intuitive leaps, this story and the sequel are fascinating. Mayer’s assumed connection between blood color and metabolic oxidation rate was certainly oversimplified and partly wrong, but this germ of a theory brought an intellectual excitement and stimulation Mayer had never before experienced. It did not take him long to see his discovery as much more than a new medical fact: metabolic oxidation was a physiological conversion process in which heat was produced from food materials, a chemical effect producing a thermal effect. Mayer was convinced that the chemical effect and the thermal effect were somehow related; to use the terminology he adopted to express his theory, the chemical reaction was a “force” that changed its form but not its magnitude in the metabolic process. And most important in Mayer’s view, this interpretation of metabolic oxidation was just one instance of a general principle.
Conservation of Force (Energy)
In 1841 Mayer, now back in Heilbronn, began a paper that summarized his point of view in the broadest terms. He wrote that “all bodies are subject to change . . . [which] cannot happen without a cause . . . [that] we call force,” that “we can derive all phenomena from a basic force,” and that “forces, like matter, are invariable.” His intention, he said, was to write physics as a science concerned with “the nature of the existence of force.” The program of this physics paralleled that of chemistry. Chemists dealt with the properties of matter, and relied on the principle that mass is conserved. Physicists should similarly study forces and adopt a principle of conservation of force. Both chemistry and physics were based on the principle that the “quantity of [their] entities is invariable and only the quality of these entities is variable.”
Mayer’s use of the term force requires some explanation. It was common for nineteenth-century physicists to give the force concept a dual meaning. They used it at times in the Newtonian sense, to denote a push or pull, but just as often the usage implied that force was synonymous with the modern term energy. The modern definition of the word “energy” the capacity to do work was not introduced until the 1850s, by William Thomson. In the above quotations, and throughout most of Mayer’s writings, it is appropriate to assume the second usage, and to read “energy” for “force.” With that simple but significant change, Mayer’s thesis becomes an assertion of the principle of the conservation of energy.
Rejection
Mayer submitted his 1841 paper to Johann Poggendorff’s Annalen der Physik und Chemie. It was not accepted for publication, or even returned with an acknowledgment. But, according to one of Mayer’s biographers, R. Bruce Lindsay, the careless treatment was a blessing in disguise. Mayer’s detailed arguments in the paper were “based on a profound misunderstanding of mechanics.” Although the rejection was a blow to Mayer’s pride, “it was a good thing for [his] subsequent reputation that [the paper] did not see the light of day.”
If Mayer had great pride, he had even more perseverance. With help from his friend Carl Baur (later a professor of mathematics in Stuttgart), he improved the paper, expanded it in several ways, and at last saw it published in Justus von Liebig’s Annalen der Chemie und Pharmacie in 1842. Mayer’s most important addition to the paper was a calculation of the mechanical effect, work done in the expansion of a gas, produced by a thermal effect, the heating of the gas. This was an evaluation of the “mechanical equivalent of heat,” a concern independently occupying Joule at about the same time. Whether or not Mayer made the first such calculation became the subject of a celebrated controversy. One thing that weakened Mayer’s priority claim was that he omitted all details but the result in his calculation in the 1842 paper. Not until 1845, in a more extended paper, did he make his method clear. By 1845, Joule was reporting impressive experimental measurements of the mechanical equivalent of heat.
In the 1842 paper, Mayer based his ultimately famous calculation on the experimental fact that it takes more heat to raise the temperature of a gas held at constant pressure than at constant volume. Mayer could see in the difference between the constant-pressure and constant-volume results a measure of the heat converted to an equivalent amount of work done by the gas when it expands against constant pressure. He could also calculate that work, and the work to heat ratio, was a numerical evaluation of the mechanical equivalent of heat. His calculation showed that 1 kilocalorie of heat converted to work could lift 1 kilogram 366 meters. In other words, the mechanical equivalent of heat found by Mayer was 366 kilogram-meters per kilocalorie.
This was the quantity Joule had measured, or was about to measure, in a monumental series of experiments started in 1843. Joule’s best result (labeled as it was later with a J ) was
J = 425 kilogram-meters per kilocalorie.
Mayer’s calculation was incorrect principally because of errors in heat measurements. More-accurate measurements by Victor Regnault in the 1850s brought Mayer’s calculation much closer to Joule’s result,
J = 426 kilogram-meters per kilocalorie.
In addition to clarifying his determination of the mechanical equivalent of heat, Mayer’s 1845 paper also broadened his speculations concerning the conservation of energy, or force, as Mayer’s terminology had it. Two quotations will show how committed Mayer had become to the conservation concept: “What chemistry performs with respect to matter, physics has to perform in the case of force. The only mission of physics is to become acquainted with force in its various forms and to investigate the conditions governing its change. The creation or destruction of force, if [either has] any meaning, lies outside the domain of human thought and action.” And: “In truth there exists only a single force. In never-ending exchange this circles through all dead as well as living nature. In the latter as well as the former nothing happens without form variation of force!”
Mayer submitted his 1845 paper to Liebig’s Annalen; it was rejected by an assistant editor, apparently after a cursory reading. The assistant’s advice was to try Poggendorff’s Annalen, but Mayer did not care to follow that publication route again. In the end, he published the paper privately, and hoped to gain recognition by distributing it widely. But beyond a few brief journal listings, the paper, Mayer’s magnum opus, went unnoticed.
Over the Edge and Back
Although by this time Mayer was losing ground in his battle against discouragement, perseverance still prevailed. In 1846, he wrote another paper (this one, on celestial mechanics, anticipated work done much later by William Thomson), and again had to accept private publication. Professional problems were now compounded by family and health problems. During the years 1846 to 1848, three of Mayer’s children died, and his marriage began to deteriorate. Finally, in 1850, he suffered a nearly fatal breakdown. An attack of insomnia drove him to a suicide attempt; the attempt was unsuccessful, but from the depths of his despair Mayer might have seen this as still another failure.
In an effort to improve his condition, Mayer voluntarily entered a sanatorium. Treatment there made the situation worse, and finally he was committed to an asylum, where his handling was at best careless and at times brutal. The diagnosis of his mental and physical condition became so bleak that the medical authorities could offer no hope, and he was released from the institution in 1853.
It may have been Mayer’s greatest achievement that he survived, and even partially recovered from, this appalling experience. After his release, he returned to Heilbronn, resumed his medical practice in a limited way, and for about ten years deliberately avoided all scientific activity. In slow stages, and with occasional relapses, his health began to return. That Mayer could, by an act of will it seems, restore himself to comparatively normal health, demonstrated, if nothing else did, that his mental condition was far from hopelessly unbalanced. To abandon entirely for ten years an effort that had become an obsession was plainly an act of sanity.
The period of Mayer’s enforced retirement, the 1850s, was a time of great activity in the development of thermodynamics. Energy was established as a concept, and the energy conservation principle was accepted by most theorists. This work was done mostly by James Joule in England, by Rudolf Clausius in Germany, and by William Thomson and Macquorn Rankine in Scotland, with little appreciation of Mayer’s efforts. Not only was Mayer’s theory ignored during this time, but in 1858 Mayer himself was reported by Liebig to have died in an asylum. Protests from Mayer did not prevent the appearance of his official death notice in Poggendorff’s Handworterbuch.
Strange Success
The final episode in this life full of ironies will seem like the ultimate irony. Recognition of Mayer’s achievements finally came, but hardly in a way deserved by a man who had endured indifference, rejection, breakdown, cruel medical treatment, and reports of his own death. In the early 1860s Mayer, now peacefully tending his vineyards in Heilbronn, suddenly became the center of a famous scientific controversy.
It all started when John Tyndall, a popular lecturer, professor, and colleague of Michael Faraday at the Royal Institution in London, prepared himself for a series of lectures on heat. He wrote to Hermann Helmholtz and Rudolf Clausius in Germany for information. Included in Clausius’s response was the comment that Mayer’s writings were not important. Clausius promised to send copies of Mayer’s papers nevertheless, and before mailing the papers he read them, apparently for the first time with care. Clausius wrote a second letter with an entirely different assessment: “I must retract the statements in my last letter that you would not find much of importance in Mayer’s writings; I am astonished at the multitude of beautiful and correct thoughts which they contain.” Clausius was now convinced that Mayer had been one of the first to understand the energy concept and its conservation doctrine. Helmholtz also sent favorable comments on Mayer, pointing especially to the early evaluation of the mechanical equivalent of heat.
Tyndall was a man who loved controversy and hated injustice. Because his ideas concerning the latter were frequently not shared by others who were equally adept in the practice of public controversy, he was often engaged in arguments that were lively, but not always friendly. When Tyndall decided to be Mayer’s champion, he embarked on what may have been the greatest of all his controversies. As usual, he chose as his forum the popular lectures at the Royal Institution. He had hastily decided to broaden his topic from heat to the general subject of energy, which was by then, in the 1860s, mostly understood; the title of his lecture was “On Force.” (Faraday and his colleagues at the Royal Institution still preferred to use the term “force” when they meant “energy.”)
Tyndall began by listing many examples of energy conversion and conservation, and then summarized Mayer’s role with the pronouncement, “All that I have brought before you has been taken from the labors of a German physician, named Mayer.” Mayer should, he said, be recognized as one of the first thermodynamicists, “a man of genius arriving at the most important results some time in advance of those whose lives were entirely devoted to Natural Philosophy.” Tyndall left no doubt that he felt Mayer had priority claims over Joule: “Mr. Joule published his first paper ‘On the Mechanical Value of Heat’ in 1843, but in 1842 Mayer had actually calculated the mechanical equivalent of heat.” In the gentlemanly world of nineteenth century scientific discourse, this was an invitation to verbal combat. It brought quick responses from Joule and Thomson, and also from Thomson’s close friend Peter Guthrie Tait, professor of natural philosophy at the University of Edinburgh, and Tyndall’s match in the art of polemical debate.
Joule was the first to reply, in a letter published in the Philosophical Magazine. He could not, he said, accept the view that the “dynamical theory of heat” (that is, the theory of heat that, among other things, was based on the heat-work connection) was established by Mayer, or any of the other authors who speculated on the meaning of the conversion processes. Reliable conclusions “require experiments,” he wrote, “and I therefore fearlessly assert my right to the position which has been generally accorded to me by my fellow physicists as having been the first to give decisive proof of the correctness of this theory.”
Tyndall responded to Joule in another letter to the Philosophical Magazine, protesting that he did not wish to slight Joule’s achievements: “I trust you will find nothing [in my remarks] which indicates a desire on my part to question your claim to the honour of being the experimental demonstrator of the equivalence of heat and work.” Tyndall was willing to let Mayer speak for himself; at Tyndall’s suggestion, Mayer’s papers on the energy theme were translated and published in the Philosophical Magazine.
But this did not settle the matter. An article with both Thomson and Tait listed as authors (although the style appears to be that of Tait) next appeared in a popular magazine called Good Words, then edited by Charles Dickens. In it, Mayer’s 1842 paper was summarized as mainly a recounting of previous work with a few suggestions for new experiments; “a method for finding the mechanical equivalent of heat [was] propounded.” This was, the authors declared, a minor achievement, and they could find no reason to surrender British claims:
On the strength of this publication an attempt has been made to claim for Mayer the credit of being the first to establish in all its generality the principle of the Conservation of Energy. It is true that la science n’a pas de patrie and it is highly creditable to British philosophers that they have so liberally acted according to this maxim. But it is not to be imagined that on this account there should be no scientific patriotism, or that, in our desire to do justice to a foreigner, we should depreciate or suppress the claims of our countrymen.
Tyndall replied, again in the Philosophical Magazine, pointedly directing his remarks to Thomson alone, and questioning the wisdom of discussing weighty matters of scientific priority in the pages of a popular magazine. He now relaxed his original position and saw Joule and Mayer more in a shared role:
Mayer’s labors have in some measure the stamp of profound intuition, which rose, however, to the energy of undoubting conviction in the author’s mind. Joule’s labours, on the contrary, are in an experimental demonstration. True to the speculative instinct of his country, Mayer drew large and weighty conclusions from slender premises, while the Englishman aimed, above all things, at the firm establishment of facts. And he did establish them. The future historian of science will not, I think, place these men in antagonism.
Tait was next heard from. He wrote to one of the editors of the Philosophical Magazine, first offering the observation that if Good Words was not a suitable medium for the debate of scientific matters, neither were certain popular lecture series at the Royal Institution. He went on: “Prof. Tyndall is most unfortunate in the possession of a mental bias which often prevents him . . . from recognizing the fact that claims of individuals whom he supposes to have been wronged have, before his intervention, been fully ventilated, discussed, and settled by the general award of scientific men. Does Prof. Tyndall know that Mayer’s paper has no claim to novelty or correctness at all, saving this, that by a lucky analogy he got an approximation to a true result from an utterly false analogy?”
Even if the polemics had been avoided, any attempt to resolve Joule’s and Mayer’s conflicting claims would have been inconclusive. If the aim of the debate was to identify once and for all the discoverer of the energy concept, neither Joule nor Mayer should have won the contest. The story of the energy concept does not end, nor does it even begin, with Mayer’s speculations and Joule’s experimental facts. Several of Kuhn’s simultaneous discoverers were earlier, although more tentative, than Joule and Mayer. In the late 1840s, after both men had made their most important contributions, the energy concept was still only about half understood; the modern distinction between the terms force and energy had not even been made clear. Helmholtz, Clausius, and Thomson still had fundamentally important contributions to make. Those who spend their time fighting priority wars should forget their individual claims and learn to appreciate a more important aspect of the sociology of science: that the scientific community, with all its diversity cutting across race, class, and nationality, can, as often as it does, arrive at a consensus acceptable to all. The final judgment in the Joule-Mayer controversy teaches this lesson. In 1870, almost a decade after the last Tyndall or Tait outburst, the Royal Society awarded its prestigious Copley medal to Joule and a year later to Mayer.
الاكثر قراءة في علماء الفيزياء
اخر الاخبار
اخبار العتبة العباسية المقدسة
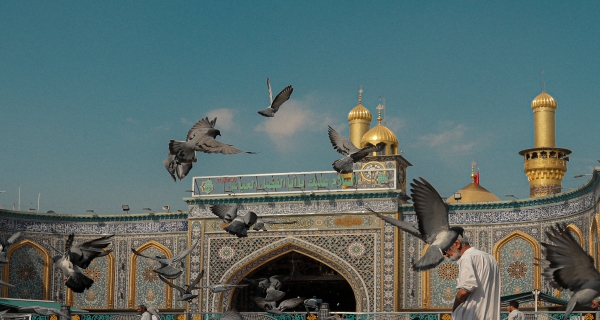
الآخبار الصحية
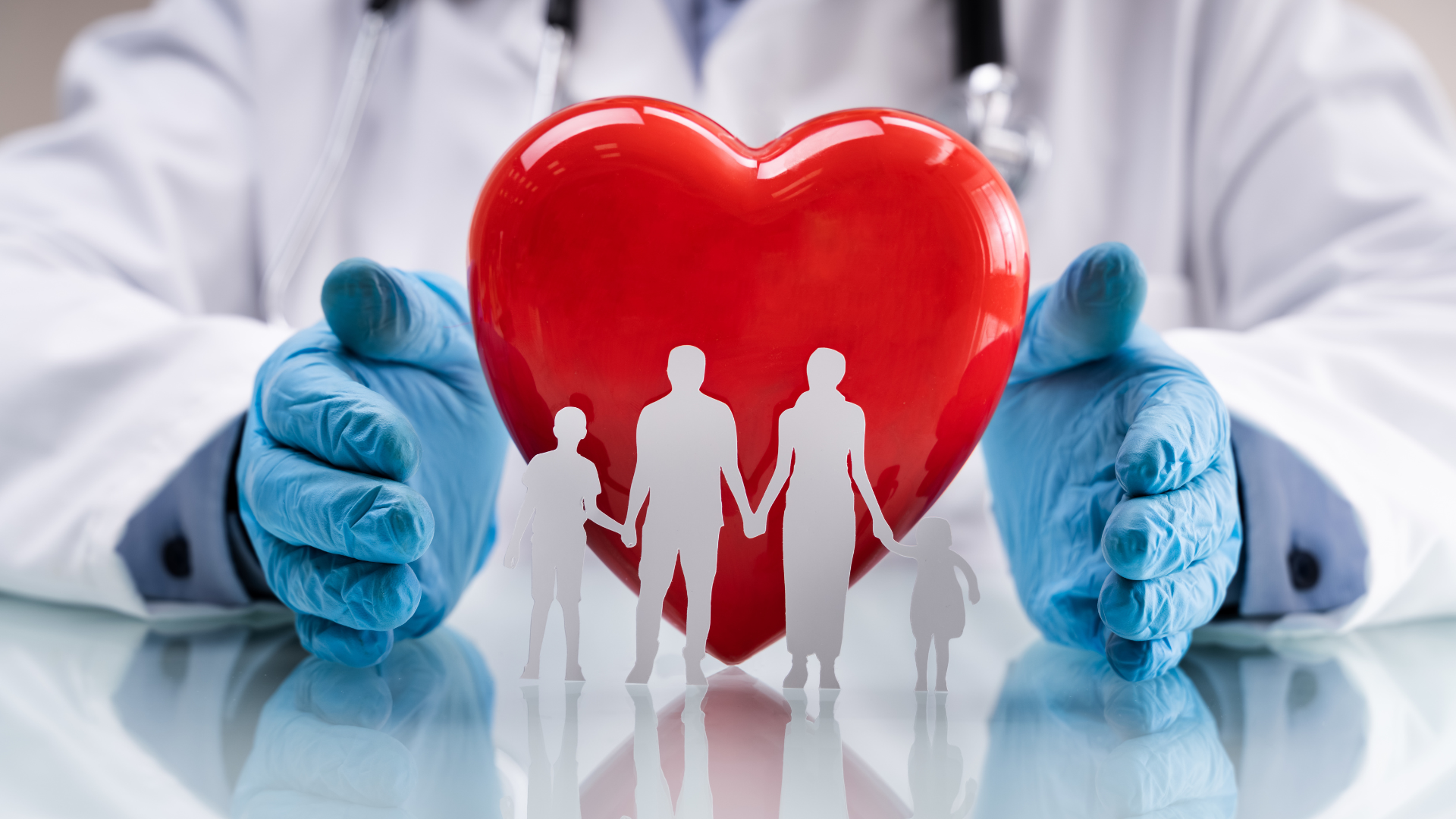